Introduction to waves
Intros
Lessons
Free to Join!
Easily See Your Progress
We track the progress you've made on a topic so you know what you've done. From the course view you can easily see what topics have what and the progress you've made on them. Fill the rings to completely master that section or mouse over the icon to see more details.Make Use of Our Learning Aids
Earn Achievements as You Learn
Make the most of your time as you use StudyPug to help you achieve your goals. Earn fun little badges the more you watch, practice, and use our service.Create and Customize Your Avatar
Play with our fun little avatar builder to create and customize your own avatar on StudyPug. Choose your face, eye colour, hair colour and style, and background. Unlock more options the more you use StudyPug.
Topic Notes
Introduction to Waves
Welcome to our exciting journey into the world of waves! We'll kick off with an engaging introduction video that sets the stage for our exploration. This video is crucial as it provides a visual foundation for understanding waves in various contexts. Throughout this lesson, we'll delve into the fascinating realm of waves, starting with a clear definition of what waves are. We'll then explore the different types of waves you encounter in everyday life and in scientific studies. Importantly, we'll focus on the two main categories of waves, which will help you classify wave behavior better. Whether you're seeing waves at the beach, hearing sound waves, or studying light waves, this lesson will equip you with the knowledge to recognize and appreciate waves in all their forms. So, let's dive in and ride the wave of learning together!
Understanding sound waves is essential for grasping the principles of acoustics. Additionally, being able to classify wave behavior at different boundaries will enhance your comprehension of wave interactions. This knowledge is not only fundamental in physics but also has practical applications in various fields such as engineering, medicine, and environmental science.
Definition and Basic Concepts of Waves
Waves are fascinating phenomena that play a crucial role in our everyday lives, from the ripples in a puddle to the sound waves that allow us to communicate. At its core, a wave is a disturbance that travels through matter, transferring energy from one point to another. This fundamental concept is essential to understanding the wave definition and how waves function in various mediums.
One of the most important aspects of waves is that they transfer energy, not matter. This energy transfer occurs without the actual movement of particles from one place to another. To illustrate this concept, let's consider the example of water waves in a puddle. When you drop a pebble into a puddle, you'll observe ripples spreading outward from the point of impact. These ripples are waves carrying energy across the surface of the water. However, the water molecules themselves don't travel with the wave; they merely oscillate up and down in place.
To further understand the structure of waves, we can examine a simple rope wave. Imagine holding one end of a rope and giving it a quick up-and-down motion. This action creates a wave that travels along the length of the rope. As the wave moves, you'll notice alternating high points and low points. The highest point of the wave is called the crest, while the lowest point is known as the trough. These terms are essential in describing the anatomy of a wave and are applicable to all types of waves, including water waves, sound waves, and even electromagnetic waves.
The rope wave example also helps demonstrate how waves transfer energy without transferring matter. As the wave travels along the rope, each section of the rope moves up and down, but the rope itself doesn't move horizontally. The energy from your initial motion is what propagates through the rope, causing each successive section to rise and fall in turn. This principle applies to all types of waves, showcasing the remarkable ability of waves to transport energy across distances without the need for matter to move along with it.
Understanding the wave definition and its properties is crucial in various fields of science and engineering. From studying seismic waves to developing telecommunications technology, the principles of wave motion and energy transfer are fundamental. By grasping these concepts, we can better appreciate the intricate ways in which waves shape our world and enable countless technological advancements.
In conclusion, waves are disturbances that propagate through matter, transferring energy without transferring the matter itself. Whether we're observing ripples in a puddle or analyzing complex electromagnetic waves, the basic principles remain the same. The concepts of crests, troughs, and energy transfer are key to understanding how waves function and impact our daily lives. As we continue to explore and harness the power of waves, their importance in science, technology, and our understanding of the natural world only grows.
Types of Waves: Mechanical and Electromagnetic
Waves are fundamental phenomena in nature, playing crucial roles in various aspects of our daily lives. They can be broadly categorized into two main types: mechanical waves and electromagnetic waves. Understanding the differences between these wave types is essential for grasping many scientific concepts and technological applications.
Mechanical waves are disturbances that propagate through a medium, transferring energy from one point to another. These waves require a physical substance to travel through, such as air, water, or solid materials. A prime example of mechanical waves is sound waves. When we speak or play an instrument, we create vibrations in the air molecules, which then propagate as sound waves. These waves travel through the air, reaching our ears and allowing us to perceive sound.
The dependence of mechanical waves on a medium explains why space is often referred to as silent. For instance, the moon is called a "silent planet" because it lacks an atmosphere. Without air molecules to transmit sound waves, no sound can travel on the moon's surface. This is why astronauts on lunar missions had to rely on radio communications rather than their voices to communicate with each other.
In contrast, electromagnetic waves do not require a medium to propagate. These waves can travel through a vacuum, making them fundamentally different from mechanical waves. Visible light is a well-known example of electromagnetic waves. Light from the sun travels millions of miles through the vacuum of space before reaching Earth. Other forms of electromagnetic waves include radio waves, microwaves, X-rays, and gamma rays.
The distinction between mechanical and electromagnetic waves can be dramatically demonstrated through the bell jar experiment. In this classic scientific demonstration, an electric bell is placed inside a glass jar connected to a vacuum pump. Initially, with air in the jar, the bell's sound can be heard clearly. As the air is gradually pumped out, creating a vacuum, the sound becomes fainter until it disappears entirely, even though the bell continues to vibrate visibly.
This experiment illustrates that sound waves, being mechanical waves, require a medium (in this case, air) to propagate. As the air is removed, the sound waves have no means of transmission, resulting in silence. However, light from the bell can still be seen through the glass, as electromagnetic waves do not need a medium to travel.
Understanding the properties of mechanical and electromagnetic waves has profound implications in various fields. In telecommunications, for example, we rely on electromagnetic waves to transmit information wirelessly over long distances. Radio and television broadcasts, mobile phone communications, and satellite transmissions all utilize electromagnetic waves.
On the other hand, the behavior of mechanical waves is crucial in fields such as seismology, where scientists study the propagation of seismic waves through the Earth to understand its internal structure and predict earthquakes. In medicine, ultrasound imaging uses high-frequency sound waves to create images of internal body structures, relying on the principles of mechanical wave propagation.
The study of waves also extends to more complex phenomena, such as the wave-particle duality in quantum mechanics, where particles can exhibit wave-like properties and vice versa. This concept has revolutionized our understanding of the fundamental nature of matter and energy.
In conclusion, the distinction between mechanical and electromagnetic waves is a fundamental concept in physics with far-reaching applications. While mechanical waves like sound require a medium to propagate, electromagnetic waves like light can travel through a vacuum. This difference not only explains phenomena we observe in our daily lives but also forms the basis for numerous technological advancements and scientific discoveries. As we continue to explore and harness the properties of waves, our understanding of the universe and our ability to innovate in fields ranging from communications to medical imaging will undoubtedly expand.
Electromagnetic Spectrum
The electromagnetic spectrum is a fascinating concept that encompasses a wide range of energy waves traveling through space. These waves are a fundamental part of our universe and play crucial roles in various aspects of our lives, from communication to medical imaging. The electromagnetic spectrum includes different types of electromagnetic waves, each with unique properties and applications.
Starting from the highest energy waves, we have gamma rays. These are the most energetic waves in the spectrum and are often associated with radioactive decay and cosmic events. Next are X-rays, which are commonly used in medical imaging to see inside the human body. Moving down the spectrum, we encounter ultraviolet (UV) rays, which are responsible for sunburns but also play a role in sterilization processes.
Visible light, the part of the spectrum we can see with our eyes, comes next. This narrow band of wavelengths allows us to perceive colors and is essential for photosynthesis in plants. Just beyond visible light, we find infrared waves, which we experience as heat. Infrared technology is used in thermal imaging and remote controls.
Microwaves, best known for their use in cooking, are next in the spectrum. These waves are also crucial for satellite communications and radar systems. Finally, at the lowest energy end of the spectrum, we have radio waves. These are used for broadcasting, wireless communications, and even studying distant objects in space.
One of the most remarkable properties of electromagnetic waves is their ability to travel through a vacuum. Unlike sound waves, which require a medium like air or water to propagate, electromagnetic waves can move through empty space. This property allows us to receive light from distant stars and use radio waves for long-distance communication.
It's important to note that while we've introduced the different types of waves in the electromagnetic spectrum, there's much more to explore. Future discussions will delve into key concepts such as wavelength and frequency, which help distinguish between these different types of waves and explain their unique behaviors and applications. Understanding these properties is crucial for grasping the full scope and importance of the electromagnetic spectrum in science and technology.
Categories of Waves: Transverse and Longitudinal
Waves are fundamental phenomena in nature, playing crucial roles in various physical processes. Understanding the two main categories of waves - transverse and longitudinal - is essential for grasping the behavior of energy propagation in different mediums. This article delves into the characteristics of these wave types, their differences, and their applications in both mechanical and electromagnetic contexts.
Transverse Waves: Perpendicular Motion
Transverse waves are characterized by particle motion that occurs perpendicular to the direction of wave propagation. In simpler terms, the oscillations or vibrations of the medium's particles move up and down (or side to side) while the wave itself travels horizontally. A classic example of a transverse wave is a wave on a string or rope. When you shake one end of a rope up and down, you create a wave that travels along the rope's length, but the individual particles of the rope move perpendicular to this direction.
Longitudinal Waves: Parallel Motion
In contrast, longitudinal waves involve particle motion that is parallel to the direction of wave propagation. The particles of the medium move back and forth along the same line as the wave's travel. Sound waves in air are a prime example of longitudinal waves. As sound propagates through the air, it creates areas of compression (where air molecules are pushed together) and rarefaction (where air molecules are spread apart), but these compressions and rarefactions occur in the same direction as the wave's movement.
Illustrating the Difference
To visualize the difference between transverse and longitudinal waves, imagine a slinky toy:
- For a transverse wave, hold the slinky horizontally and move one end up and down. The wave will travel along the slinky's length, but each coil will move up and down.
- For a longitudinal wave, push and pull one end of the slinky along its length. The wave will travel through the slinky as compressions and expansions, with each coil moving back and forth in the same direction as the wave.
Mechanical vs. Electromagnetic Waves
It's important to note that both mechanical and electromagnetic waves can be categorized as either transverse or longitudinal. Mechanical waves require a medium to propagate, such as water waves or sound waves in air. Electromagnetic waves, like light or radio waves, can travel through a vacuum and are always transverse.
Examples in Nature and Technology
Transverse waves are commonly observed in:
- Water ripples on a pond's surface
- Light waves
- Electromagnetic radiation (radio waves, X-rays, etc.)
- Seismic S-waves (secondary waves in earthquakes)
Longitudinal waves are found in:
- Sound waves in air and liquids
- Compression waves in springs
- Seismic P-waves (primary waves in earthquakes)
- Ultrasound used in medical imaging
Importance in Physics and Engineering
Understanding the distinction between transverse and longitudinal waves is crucial in various fields of physics and engineering. This knowledge is applied in:
- Acoustics and sound engineering
- Seismology and earthquake prediction
- Optical fiber communications
- Medical imaging technologies
- Material science and stress analysis
By grasping the concepts of transverse and longitudinal waves, scientists and engineers can better analyze and manipulate wave behavior for various applications, from improving sound quality in concert halls to developing more efficient communication systems. The study of these wave types continues to be a fundamental
Examples and Applications of Waves
Waves are an integral part of our daily lives, playing crucial roles in various fields and technologies. Understanding wave properties and their applications is essential for scientific advancements and problem-solving across multiple disciplines. This section explores real-world examples and applications of different types of waves, demonstrating their significance in medicine, communications, and everyday life.
In the medical field, ultrasound waves have revolutionized diagnostic imaging and therapeutic procedures. Ultrasound technology uses high-frequency sound waves to create real-time images of internal organs, blood flow, and developing fetuses. This non-invasive technique is widely used in obstetrics, cardiology, and vascular studies. Additionally, focused ultrasound waves are employed in treatments such as breaking down kidney stones or targeting tumors without invasive surgery.
The realm of communications heavily relies on various types of waves. Radio waves, a form of electromagnetic radiation, are fundamental to wireless communication systems. From AM/FM radio broadcasts to cellular networks and Wi-Fi, radio waves enable the transmission of information over long distances. Television broadcasting, satellite communications, and GPS navigation systems also utilize different frequencies of radio waves to function effectively.
In our everyday lives, we constantly interact with light waves. Visible light allows us to perceive the world around us, while infrared waves are used in remote controls and thermal imaging cameras. UV waves are harnessed in sterilization processes and for vitamin D synthesis in our skin. The understanding of light wave properties has led to the development of fiber optic technology, which revolutionized telecommunications by enabling high-speed data transmission over long distances.
Sound waves are another ubiquitous form of waves in our daily experiences. Beyond enabling verbal communication and enjoying music, sound waves find applications in various fields. In oceanography, sonar technology uses sound waves to map ocean floors and detect underwater objects. The entertainment industry utilizes sound waves in surround sound systems and noise-canceling headphones. In industrial settings, ultrasonic cleaning devices employ high-frequency sound waves to remove contaminants from objects.
The study of seismic waves is crucial in geology and earthquake prediction. These waves, generated by earthquakes or explosions, travel through the Earth's layers and provide valuable information about the planet's internal structure. Seismologists analyze these waves to understand tectonic plate movements and assess potential earthquake risks.
In the field of renewable energy, wave power harnesses the energy of ocean surface waves to generate electricity. Wave energy converters capture the kinetic and potential energy of waves, offering a promising alternative to traditional power sources in coastal regions.
The principles of wave interference and diffraction find applications in various technologies. For instance, noise-canceling headphones use destructive interference to reduce ambient noise. In optics, diffraction gratings separate light into its component wavelengths, which is essential in spectroscopy and telecommunications.
Understanding wave properties is also crucial in developing advanced materials. For example, metamaterials with engineered electromagnetic properties can manipulate waves in ways not found in nature, leading to applications like invisibility cloaks and super-lenses.
In conclusion, waves permeate numerous aspects of our lives and scientific endeavors. From medical diagnostics to global communications, and from renewable energy to materials science, the applications of wave properties are vast and diverse. As our understanding of waves continues to grow, so does our ability to harness their potential in solving complex problems and developing innovative technologies across various scientific disciplines.
Conclusion
In this introduction to waves, we've explored fundamental wave concepts that form a crucial physics foundation. We defined waves as disturbances that transfer energy through matter or space without transferring mass. We examined various types of waves, including mechanical and electromagnetic, and categorized them as transverse or longitudinal based on their motion. Understanding these wave concepts is essential for further study in physics and other sciences. Waves are ubiquitous in our daily lives, from sound and light waves to water ripples and seismic activity. We encourage students to review the introduction video and explore more about waves in their surroundings. By grasping these basic principles, you'll be better equipped to tackle more advanced wave topics and their applications in fields like acoustics, optics, and quantum mechanics. Remember, wave study is not just theoretical; it has practical implications in technology, communication, and our understanding of the universe.
Introduction to Waves
Introduction to Waves
What are waves?
Step 1: Introduction to the Topic
So, hello everyone. Today's lesson is about the introduction to waves. In this session, we will cover some types of waves and fundamental information about waves. This will help us build a solid foundation for understanding the concept of waves.
Step 2: Definition of Waves
To start, we need to understand the definition of waves. A wave is defined as a disturbance that can travel through matter. For instance, if you observe water waves in a puddle, you will notice that when you drop an object into the puddle, waves travel away from the point where the object was dropped. This disturbance in the water is what we call a wave.
Step 3: Understanding the Medium
When you drop something into a puddle, you disturb the medium, which in this case is the water. The disturbance that travels through the water is the wave. It is important to note that the matter itself does not transfer; what transfers is the energy. For example, when you drop an object into the puddle, the object has potential energy and kinetic energy as it falls. This energy is then transferred to the water, causing the waves to form and move outward from the source.
Step 4: Energy Transfer
As the object falls into the puddle, its energy is transferred to the water. The water in the puddle does not move out of the puddle; instead, the energy is transferred through the water, creating waves. This means that the matter (water) does not transfer, but the energy does. This concept is crucial in understanding how waves work.
Step 5: Example with a Rope
To further illustrate the concept of energy transfer in waves, consider a rope. If you move your hand up and down while holding one end of the rope, the kinetic energy from your hand is transferred to the rope, causing it to move up and down. This movement creates a disturbance that travels through the rope. The direction in which the disturbance moves is important, as it indicates the direction of the wave.
Step 6: Direction of Wave Motion
When you move your hand up and down, the disturbance moves through the rope in the direction of the wave. This is known as the direction of motion. Understanding the direction of wave motion is essential for comprehending how waves propagate through different mediums.
Step 7: Crest and Trough
In the context of waves, it is also important to understand the terms "crest" and "trough." The crest is the highest point of the wave, while the trough is the lowest point. These terms help describe the shape and behavior of waves as they travel through a medium.
FAQs
1. What is the basic concept of waves?
Waves are disturbances that transfer energy through matter or space without transferring mass. They can be mechanical (requiring a medium) or electromagnetic (can travel through vacuum). Waves are characterized by properties such as amplitude, wavelength, frequency, and speed.
2. What are the 4 main types of waves?
The four main types of waves are: 1. Water waves (ocean waves, ripples) 2. Sound waves 3. Light waves 4. Electromagnetic waves (radio waves, X-rays, gamma rays, etc.)
3. How do you introduce waves?
To introduce waves, start with everyday examples like ocean waves or sound. Explain that waves transfer energy without transferring matter. Demonstrate with a slinky or rope to show wave motion. Introduce key terms like crest, trough, wavelength, and amplitude. Discuss the difference between transverse and longitudinal waves.
4. What are the two main categories of waves?
The two main categories of waves are: 1. Mechanical waves: Require a medium to propagate (e.g., sound waves, water waves) 2. Electromagnetic waves: Can travel through vacuum (e.g., light, radio waves, X-rays)
5. How do transverse and longitudinal waves differ?
Transverse waves have particle motion perpendicular to the direction of wave propagation (e.g., light waves, waves on a string). Longitudinal waves have particle motion parallel to the direction of wave propagation (e.g., sound waves, compression waves in springs). The key difference is in the direction of particle oscillation relative to wave travel.
Prerequisite Topics
Before diving into the fascinating world of waves, it's crucial to understand the foundational concepts that pave the way for a comprehensive grasp of wave phenomena. One of the most important prerequisite topics for an introduction to waves is wave behavior at boundaries. This fundamental concept is essential because it provides insight into how waves interact with different mediums and obstacles, which is a cornerstone of wave physics.
Understanding wave behavior at boundaries is critical for several reasons. Firstly, it helps explain many everyday phenomena we observe, such as the reflection of light off surfaces or the refraction of light through water. These principles are not only applicable to light waves but also to sound waves, water waves, and even electromagnetic waves.
When studying an introduction to waves, knowledge of wave behavior at boundaries allows students to predict and analyze how waves will react when they encounter different materials or interfaces. This understanding is fundamental to grasping more complex wave concepts, such as interference patterns, diffraction, and wave superposition.
Moreover, the principles learned from wave behavior at boundaries have numerous practical applications. In fields like optics, acoustics, and telecommunications, engineers and scientists rely on these concepts to design everything from optical fibers to noise-canceling headphones. Without a solid foundation in how waves interact with boundaries, it would be challenging to comprehend these advanced applications.
As students progress in their study of waves, they'll find that the concepts from wave behavior at boundaries continually resurface. Whether discussing the propagation of seismic waves through the Earth's layers or the functioning of fiber-optic cables, the principles remain the same. This prerequisite topic serves as a building block for understanding more complex wave phenomena and their real-world implications.
In conclusion, mastering wave behavior at boundaries is not just about memorizing formulas or concepts. It's about developing a intuitive understanding of how waves interact with their environment. This knowledge forms the foundation for exploring the vast and exciting field of wave physics. As students embark on their journey to understand waves, they'll find that this prerequisite topic is an indispensable tool in their physics toolkit, enabling them to unravel the complexities of wave phenomena in nature and technology.
In this lesson, we will learn:
- What are waves?
- Mechanical Waves VS. Electromagnetic Waves Vs. Surface Waves
- Transverse Waves VS. Longitudinal Waves
Notes:
- Definition of wave
Rhythmic disturbances that can travel through matter. (The matter does not transfer). Energy is transported from one location to another.
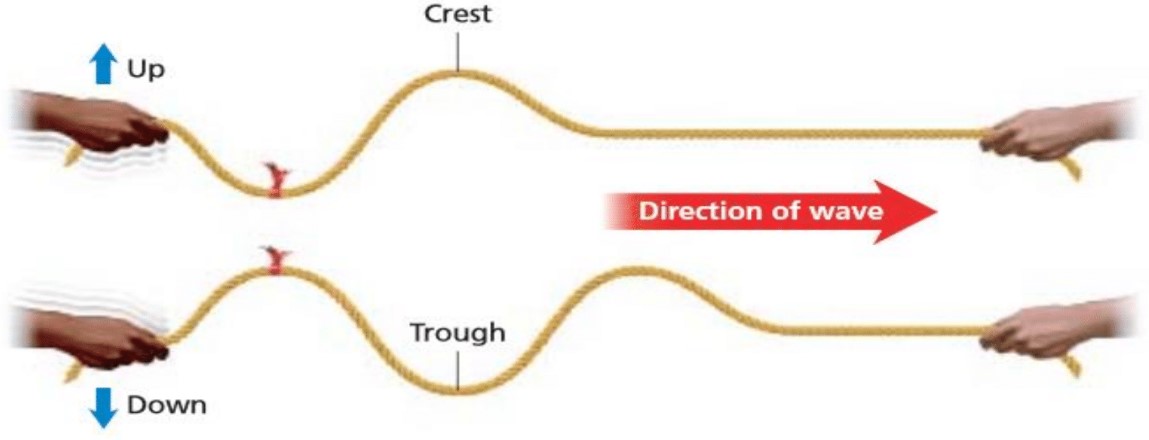
- Mechanical Waves VS. Electromagnetic Waves
- Mechanical Waves
Mechanical waves need medium (substance) to transfer, Example; Sound waves, Water waves. - Electromagnetic Waves
Electromagnetic waves do not need medium to transfer, they can travel trough vacuum, Example; Radio waves, Microwaves, Infrared, visible light, ultraviolet, X-rays, and Gamma rays.
- Mechanical Waves
- Transverse Waves VS. Longitudinal Waves Vs. Surface Waves
- Transverse Waves
Direction of motion of particles is PERPENDICULAR to the direction of motion of wave.
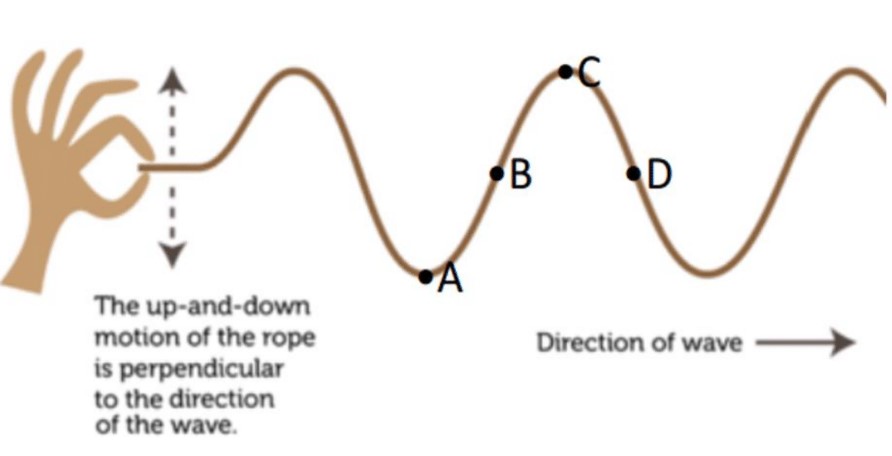
- Longitudinal Waves
Direction of motion of particles is PARALLEL to the direction of motion of waves.
Note: All electromagnetic waves are transverse waves, for example: radio waves, x-rays
Note: Some mechanical waves are transverse waves, for example: water waves, vibrating spring
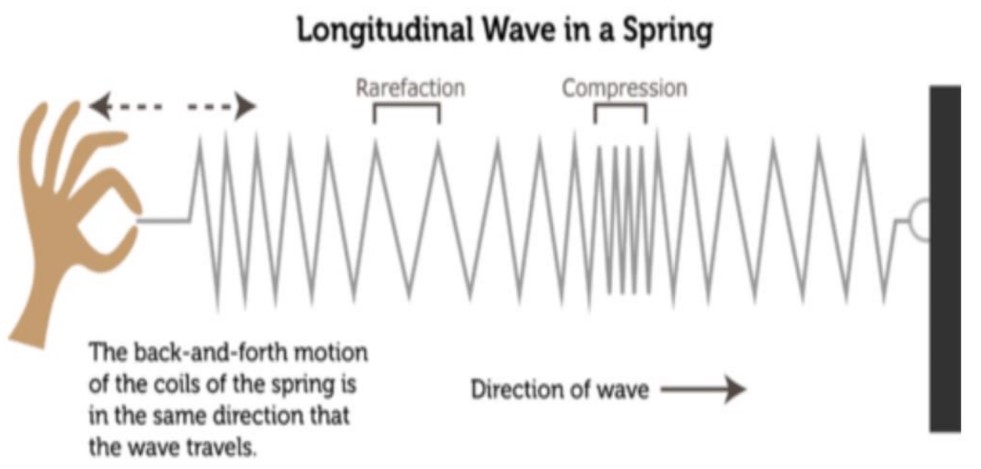
- Surface Waves
They have the characteristics of both longitudinal and transverse waves.
Example: ocean waves are longitudinal, at the surface, but particles move in both parallel and perpendicular to the direction of wave motion.
Note: Some mechanical waves are longitudinal waves, such as: sound waves
remaining today
remaining today