In this lesson, we will learn:
- The nature of sound waves
- How to solve problems relating frequency, wavelength, velocity, pitch, and loudness.
- Physical properties of sound
- Doppler shift and applications
Notes:
- Sound is produce by vibration of molecules.
- As the result of collision of molecules, the pressure is transmitted away form the source.
- Amplitude of sound is the measure of the variation in pressure, in other words Loudness, the louder sound has higher amplitude and more energy.
- The amplitude is measured by a scale called Sound Level.
- Sound level is measured in decibels (dB).
- Pitch:the sensation of frequency, high pitch high frequency.
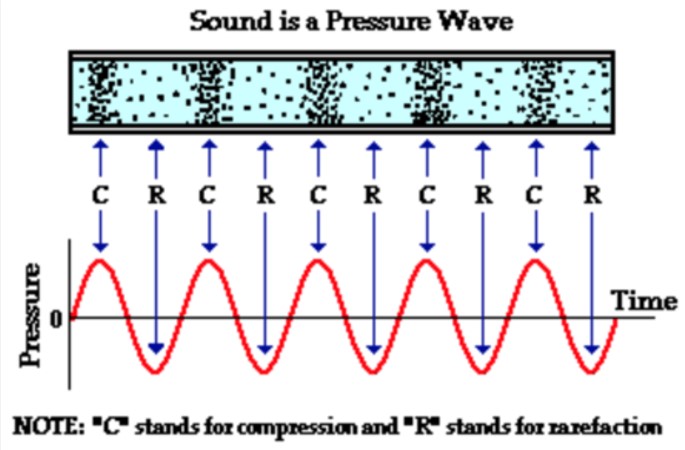
- Speed of sound wave changes upon change in temperature, the higher the temperature the higher the speed.
- Sound can travel through solids, liquids and gases. (Highest speed in solids)
- Sound can NOT travel through vacuum (No particles to move and collide).
- Sound waves are characterized as longitudinal waves.
- Echoes: Reflected sound waves.
- Doppler shift: due to motion of the sound source or the observer, the detected frequency changes. The frequency of the source does NOT change.