In this lesson, we will learn:
- Wave properties
- The relation between wave speed, wave-length, and the frequency.
- Solving exercises relating variables.
Notes:
Periodic Waves
- Pulse: Single disturbance of a medium
- Periodic Waves: Continual disturbance of a medium.
Particles in the medium move in a simple harmonic motion.
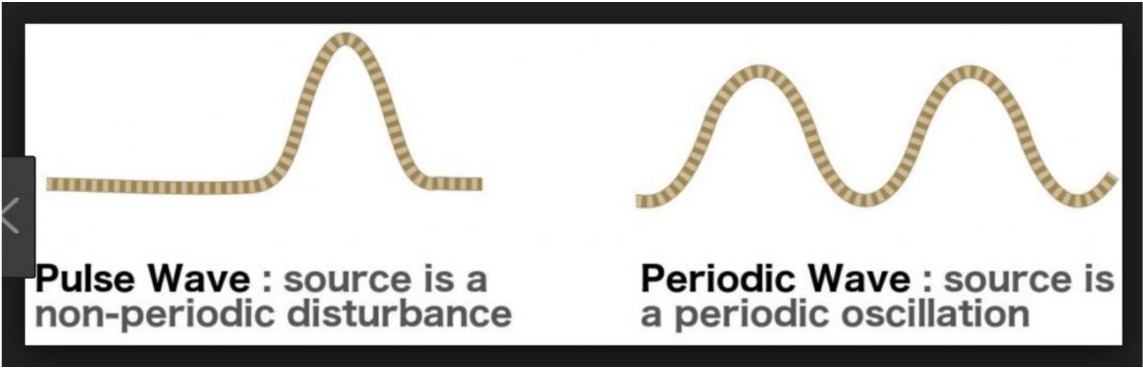
Crest: The top of the wave is called the crest; maximum displacement.
(Note: in the case of longitudinal waves we use the term Compression, representing maximum pressure or maximum density)
Trough: The bottom part of the wave is called the trough; minimum displacement.
(Note: In the case of longitudinal waves the term Rarefaction is used, minimum pressure or minimum density)
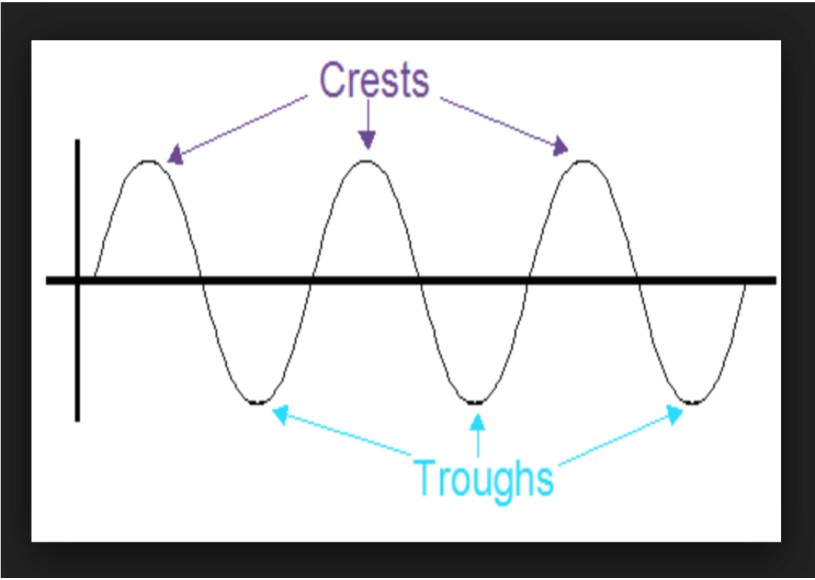
Wavelength: The distance between two successive crests (troughs) called wavelength
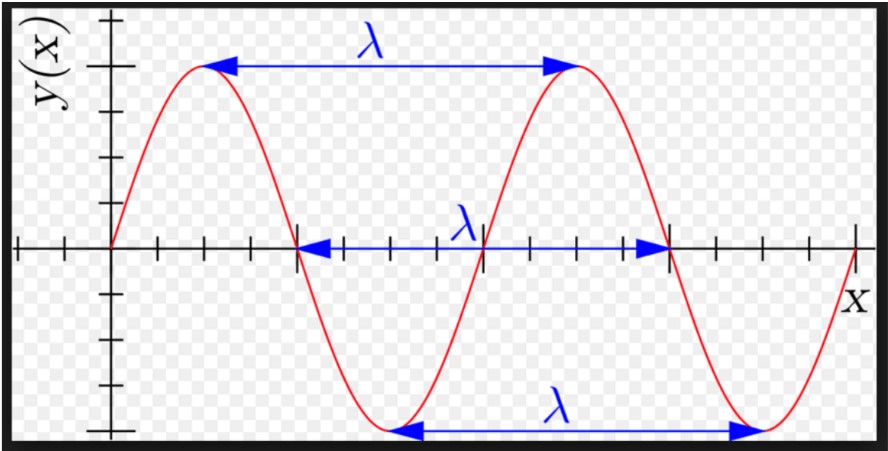
Amplitude: The height of a crest or depth of a trough called amplitude.
A high energy wave is characterized by a high?amplitude
A low energy wave is characterized by a low?amplitude
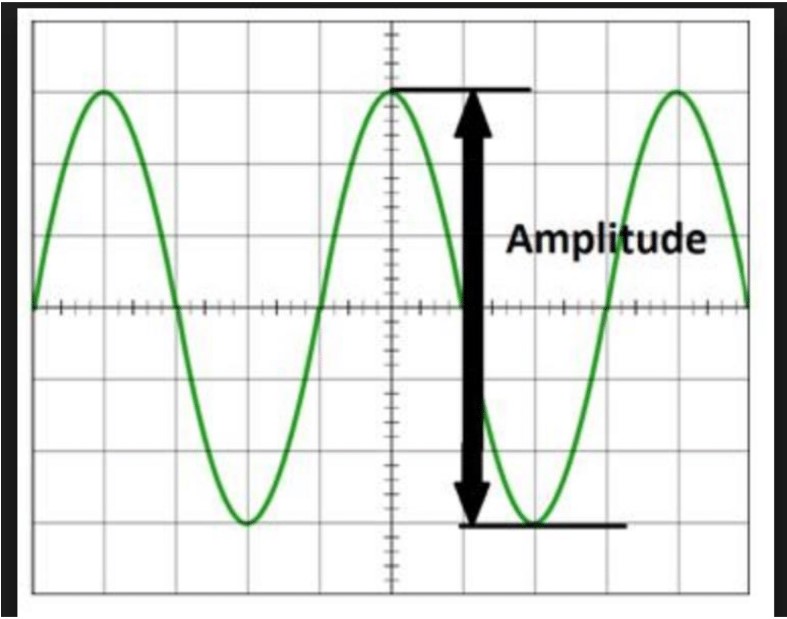
Period: The time between two consecutive crests (troughs) called the period. The period is the time taken for one wavelength to pass by a point.
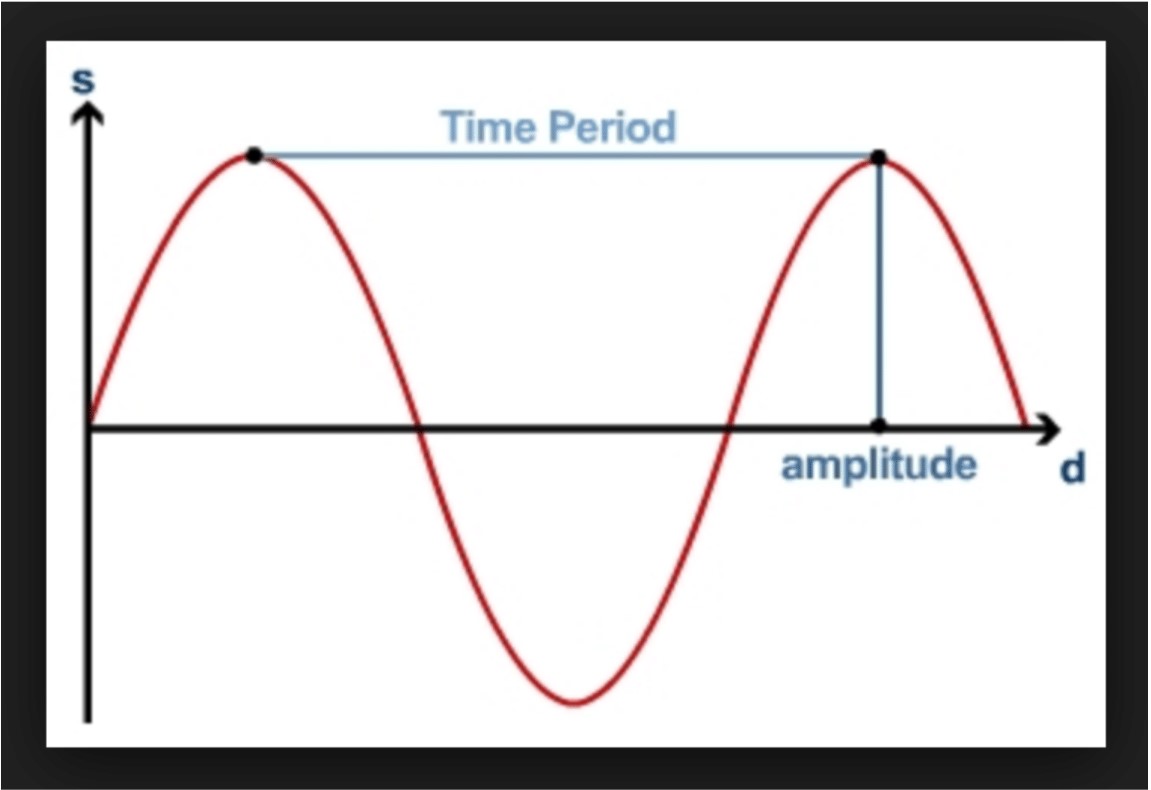
Frequency: Number of waves passing through a fixed point in ONE second.
|
|
|
|
|
|
|
|
|
|
|
|
|
|
|
Velocity: How fast the pulse is moving though a medium; the speed of the wave depends on the medium thought which it travels.
Using the kinematic equation for constant velocity, the equation for speed (velocity) of the wave is given by:
Change in position (distance between two consecutive crests) of a wave is defines as its WAVELENGTH, therefore;
Time taken between two consecutive crests is defined as the PERIOD of the motion, therefore;
Frequency is defined as number of waves per second, therefore; FREQUENCY is the reciprocal of the period,
From (1) & (2);