In this lesson, we will learn:
- Transmission Gratings
- Reflecting Gratings
- Holograms
- Resolving Power of lenses
Notes:
Transmission Gratings
- As narrow beam of light passes through a narrow slit, it shows wave light interference patterns. Light and dark bands are formed on the screen but they are kind of blended. In order to have more defined spots we use diffraction grating. A diffraction grating includes number of wholes through which we will be able to see bright spots that are separated by dark bands more clearly.
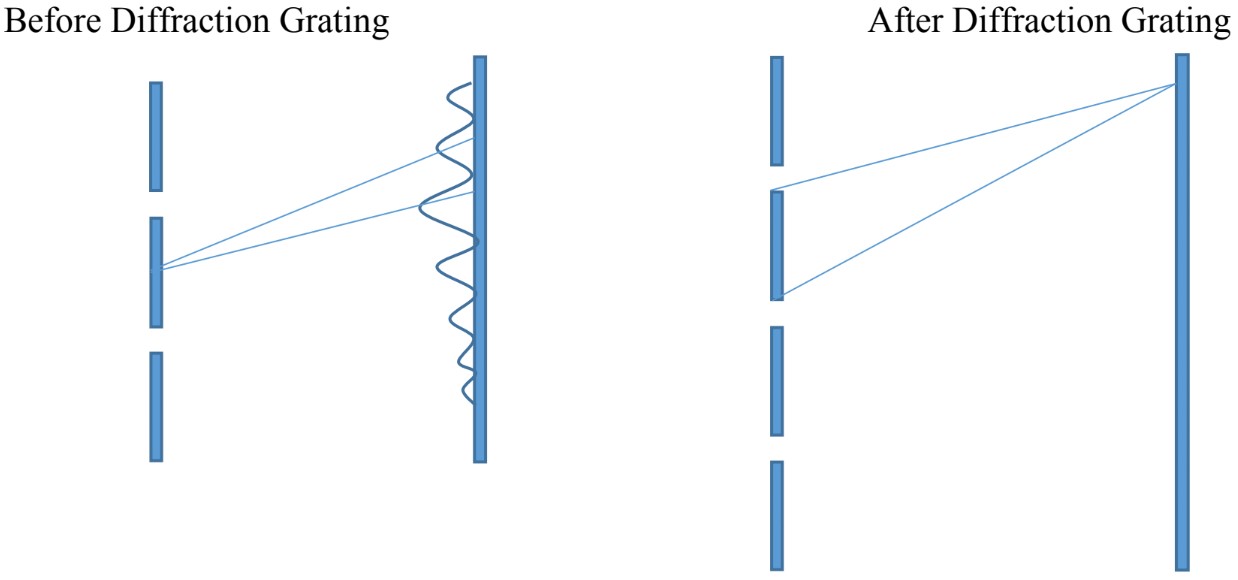
- Diffraction Gratings
- A diffraction grating is a device that transmits or reflects light and forms an interference pattern in the same way that a double slit does.
- Diffraction gratings are made by scattering very fine lines with a diamond point on glass.
- The space between the scattered lines act like slits
- Same equation as double-slit interference holds for a diffraction grating, where d is the distance between the lines.
Note: instead of measuring the distance from the central band to the first band, the angle is calculated. is so smaller than , therefore, the distance to the screen is almost equal to the perpendicular distance , so the ratio of can be replaced by sin
: Light wavelengt
: Distance between two line
: The angle between first order of bright band
Reflecting Gratings
- They are produced by scribing fine lines on metallic or reflected glass surface.
- Incoming light will be separated into different wavelengths
- Different colors are separated
- Refraction grating and interference produce the similar patterns
Holograms
- A form of Photography that produces a three-dimensional image
- (credit cards holograms)
- A coherent beam of light (laser light) is passed through a mirror and is divided into two beams
- The reference beam
- The object beam
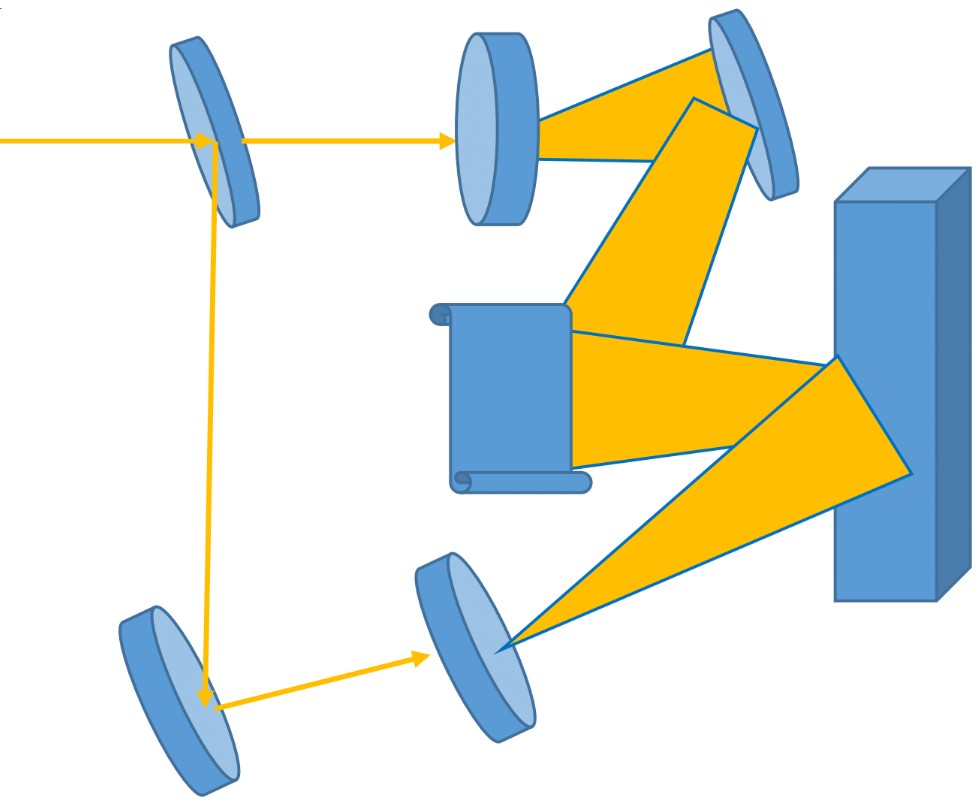
- The object beam is reflected from a mirror to illuminate the object
- The beam in turn reflects from the object into photographic film
- The reference beam is first reflected from a mirror, then it is spread by a lens and is directed over the object beam on the film
- Intensity and relative phase of the light is recorded by interference pattern
- When the film is developed the result is a hologram of the object
Resolving Power of lenses
- As light enters the lens of a telescope it passes through a circular hole, light gets diffracted.
- Diffraction limits the ability of a lens to distinguish two closely spaced object.
- The objective lens of a microscope cannot be enlarged, but the wavelength of light can be reduced.
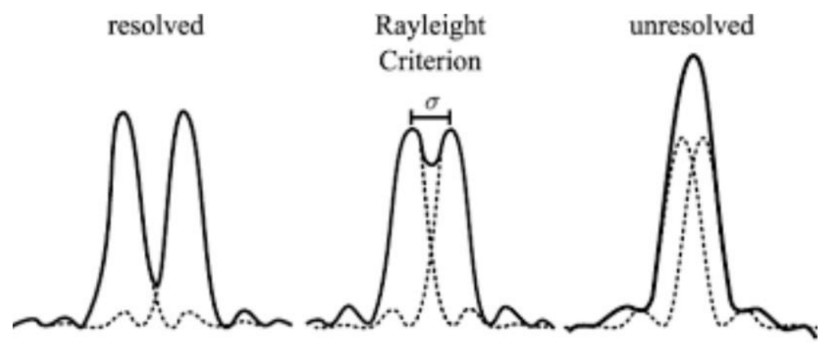
- Light coming from the stars will appear to be spread out, image looks blurry.
- If the central bright band of one star falls on the first dark band of the second one, then the two stars are seen separately and clearly.
- Increasing the size of the lens would affect the resolving power of the telescope, the Rayleigh criterion.