Metallic bonding
Intros
Lessons
Free to Join!
Easily See Your Progress
We track the progress you've made on a topic so you know what you've done. From the course view you can easily see what topics have what and the progress you've made on them. Fill the rings to completely master that section or mouse over the icon to see more details.Make Use of Our Learning Aids
Earn Achievements as You Learn
Make the most of your time as you use StudyPug to help you achieve your goals. Earn fun little badges the more you watch, practice, and use our service.Create and Customize Your Avatar
Play with our fun little avatar builder to create and customize your own avatar on StudyPug. Choose your face, eye colour, hair colour and style, and background. Unlock more options the more you use StudyPug.
Topic Notes
Introduction to Metallic Bonding
Metallic bonding is a fundamental concept in chemistry that explains the unique properties of metals. Our introduction video provides a comprehensive overview of this essential chemical bonding type, making it easier for students to grasp the concept. Metallic bonding occurs when metal atoms share their valence electrons, creating a "sea" of delocalized electrons. This electron sea is responsible for the characteristic properties of metals, such as high electrical conductivity and thermal conductivity, malleability, and ductility. Understanding metallic bonding is crucial for comprehending the behavior of everyday materials, from the aluminum in soda cans to the copper in electrical wires. The video explores how this bonding type differs from covalent and ionic bonds, and its significance in various applications. By mastering the concept of metallic bonding, students gain valuable insights into the structure and properties of materials that shape our modern world.
Structure and Features of Metallic Bonding
Metallic bonding is a unique type of chemical bonding that occurs in pure metals and alloys, giving them their distinctive properties. This bonding structure is fundamentally different from ionic and covalent bonding, and understanding it is crucial for comprehending the behavior of metals in various applications.
At the heart of metallic bonding is the arrangement of metal ions and delocalized electrons. In a metallic structure, positively charged metal ions are arranged in a regular, repeating pattern, typically in a close-packed crystal lattice. These metal ions are surrounded by a 'sea of electrons' - a cloud of freely moving valence electrons that are not bound to any specific atom.
The 'sea of electrons' model is an excellent way to visualize metallic bonding. Imagine the metal ions as buoys floating in an ocean of electrons. These electrons are highly mobile and can move freely throughout the entire metal structure. This mobility is key to many of the unique properties of metals, such as their high electrical and thermal conductivity.
In metallic bonding, the valence electrons of the metal atoms become delocalized. This means they are no longer associated with individual atoms but instead belong to the entire metallic structure. The electrostatic attraction between the positively charged metal ions and the negatively charged sea of electrons holds the structure together, creating a strong, cohesive bond.
Examples of metallic bonds can be found in all pure metals, such as copper, silver, gold, iron, and aluminum. Each of these metals exhibits the characteristic properties of metallic bonding, including malleability, ductility, and high conductivity. Alloys, which are mixtures of two or more metals or a metal with other elements, also display metallic bonding. For instance, steel (an alloy of iron and carbon) and brass (an alloy of copper and zinc) both exhibit metallic bonding characteristics.
Contrasting metallic bonding with ionic and covalent bonding helps highlight its unique features. In ionic bonding, electrons are completely transferred from one atom to another, creating oppositely charged ions held together by electrostatic forces. Covalent bonding involves the sharing of electrons between atoms, forming discrete molecules or network structures. Metallic bonding, however, involves the sharing of electrons across the entire structure, with no discrete molecules formed.
The nature of metallic bonding explains many of the characteristic properties of metals. The sea of delocalized electrons allows for easy electron flow, resulting in high electrical conductivity. The ability of these electrons to absorb and re-emit photons of various wavelengths contributes to the lustrous appearance of metals. The non-directional nature of metallic bonds, unlike the directional covalent bonds, allows metal atoms to slide past one another when stress is applied, explaining the malleability and ductility of metals.
It's important to note that metallic bonding occurs not only in pure metal samples but also in alloys. In alloys, the sea of electrons is shared among different types of metal ions, or in some cases, between metal ions and non-metal atoms. This sharing can lead to enhanced properties, which is why alloys are often preferred in various applications over pure metals.
The strength of metallic bonds can vary depending on factors such as the number of valence electrons available and the size of the metal ions. Generally, metals with more valence electrons to contribute to the electron sea form stronger metallic bonds. This is why transition metals, with their multiple valence electrons, often have higher melting points and are more durable than alkali metals.
In conclusion, metallic bonding is a fascinating and unique type of chemical bonding that gives metals their characteristic properties. The arrangement of metal ions in a sea of delocalized electrons creates a strong, cohesive structure that explains the conductivity, malleability, and ductility of metals and alloys. Understanding metallic bonding is crucial for materials science, engineering, and many technological applications that rely on the unique properties of metals.
Properties of Metals Explained by Metallic Bonding
Metallic bonding is a unique type of chemical bonding that explains the distinctive properties of metals. This bonding structure is responsible for the common characteristics shared by metallic elements, including high melting and boiling points, malleability, ductility, and excellent electrical conductivity of metals. Understanding metallic bonding is crucial for comprehending why metals behave the way they do in various applications and environments.
At its core, metallic bonding involves the sharing of valence electrons among a lattice of positively charged metal ions. These electrons form a "sea" or "cloud" of delocalized electrons that move freely throughout the metal structure. This arrangement results in strong cohesive forces between the metal ions, contributing to the unique properties of metals.
One of the most notable properties of metals is their high melting and boiling points. This characteristic is directly related to the strength of metallic bonds. The sea of electrons creates a powerful attractive force between the positive metal ions, requiring significant energy to break these bonds. For example, tungsten, a metal widely used in light bulb filaments, has an incredibly high melting point of 3,422°C (6,192°F) due to its strong metallic bonds.
Malleability, the ability of a metal to be hammered or pressed into thin sheets without breaking, is another property explained by metallic bonding. When force is applied to a metal, the layers of atoms can slide over one another without breaking the metallic bonds. This is possible because the sea of electrons can easily rearrange itself to maintain the bonding between the metal ions. Gold, known for its extreme malleability, can be hammered into sheets as thin as 0.000013 centimeters while maintaining its structural integrity.
Similarly, ductility, the ability of a metal to be drawn into thin wires, is a result of the same principle. As the metal is stretched, the atoms can move relative to each other while the electron sea continues to hold them together. Copper, widely used in electrical wiring due to its excellent ductility, can be drawn into wires less than a hair's width without breaking.
The electrical conductivity of metals is perhaps one of their most valuable properties, and it's directly attributed to metallic bonding. The sea of delocalized electrons in metals can move freely throughout the structure when an electric potential is applied. This easy movement of electrons allows metals to conduct electricity efficiently. Silver, for instance, has the highest electrical conductivity of any element, making it ideal for specialized electronic applications.
To illustrate these properties further, let's consider some common metals and their specific characteristics:
- Iron: Known for its high melting point (1,538°C or 2,800°F) and strength, iron's metallic bonds contribute to its widespread use in construction and manufacturing.
- Aluminum: Despite its lightweight nature, aluminum exhibits excellent malleability and ductility. It can be easily shaped into thin foils or drawn into wires, making it versatile for packaging and electrical applications.
- Copper: Its high electrical conductivity, combined with good ductility, makes copper the metal of choice for electrical wiring in buildings and electronics.
- Gold: Renowned for its malleability and resistance to corrosion, gold can be hammered into extremely thin sheets used in decorative applications and electronics.
The strength of metallic bonding can vary among different metals, influencing their specific properties. For example, transition metals like iron and tungsten typically have stronger metallic bonds due to their ability to involve d-orbital electrons in bonding. This results in higher melting points and greater hardness compared to metals from the main group elements.
Understanding metallic bonding not only explains the inherent properties of pure metals but also provides insights into alloys mixtures of two or more metallic elements. Alloys often exhibit enhanced properties compared to their constituent metals, as the introduction of different-sized atoms can strengthen the overall metallic structure. Steel, an alloy of iron and carbon, demonstrates how alloying can significantly improve strength and durability while maintaining the beneficial properties of metallic bonding.
In conclusion, metallic bonding is the key to understanding the unique and valuable properties of metals. From their
Factors Affecting the Strength of Metallic Bonds
Metallic bonds are a fundamental type of chemical bonding that give metals their unique properties. The strength of these bonds is crucial in determining various characteristics of metals, such as melting points, boiling points, and mechanical properties. Two main factors significantly influence the strength of metallic bonds: the charge of the ions and the ionic radius. Understanding these factors and how they change across the periodic table trends is essential for predicting and explaining metallic bond strength trends.
The first key factor affecting metallic bond strength is the charge of the ions. In metallic bonding, metal atoms lose their outermost electrons to form a "sea" of delocalized electrons, leaving behind positively charged metal ions. The greater the charge on these ions, the stronger the electrostatic attraction between the positive ions and the electron sea, resulting in stronger metallic bonds. As we move across a period in the periodic table trends from left to right, the number of valence electrons increases, potentially leading to higher ionic charges and stronger metallic bonds.
The second crucial factor is the ionic radius, which refers to the size of the metal ions in the crystal lattice. Generally, a smaller ionic radius leads to stronger metallic bonds. This is because smaller ions can pack more closely together, increasing the electrostatic interactions between the positive ions and the electron sea. The ionic radius typically decreases across a period due to the increasing nuclear charge pulling electrons closer to the nucleus, while it increases down a group as new electron shells are added.
To illustrate these trends, let's consider examples from the third period of the periodic table: sodium (Na), magnesium (Mg), and aluminum (Al). Sodium, with its single valence electron, forms a +1 ion in metallic bonding. Magnesium, with two valence electrons, forms a +2 ion, and aluminum, with three valence electrons, forms a +3 ion. As we move from sodium to magnesium to aluminum, the ionic charge increases, leading to stronger metallic bonds.
Simultaneously, the ionic radius decreases from sodium to aluminum due to the increasing nuclear charge. This decrease in size further strengthens the metallic bonds. The combined effect of increasing charge and decreasing radius results in a significant increase in metallic bond strength across this period. This trend is reflected in the melting points of these metals: sodium melts at 98°C, magnesium at 650°C, and aluminum at 660°C.
When we look at trends down a group in the periodic table trends, the situation becomes more complex. While the ionic charge remains constant within a group, the ionic radius increases as we move down. For example, in Group 1 alkali metals, the ionic radius increases from lithium to sodium to potassium. This increase in size tends to weaken the metallic bonds. However, this effect can be partially offset by the increasing number of electrons in the electron sea, which can enhance the overall cohesive energy of the metal.
The interplay between these factors leads to some interesting trends in metallic properties. For instance, while lithium has the highest melting point among the Group 1 alkali metals due to its small size, the trend doesn't continue uniformly down the group. Sodium has a lower melting point than lithium, but potassium's melting point is even lower. This demonstrates how the increasing atomic size down the group eventually outweighs the effect of the increased number of electrons in larger atoms.
Understanding these trends in metallic bond strength is crucial for predicting and explaining various properties of metals. For example, metals with stronger metallic bonds typically have higher melting and boiling points, greater hardness, and higher tensile strength. They also tend to be less reactive and have lower electrical resistivity. These properties make metals with strong metallic bonds, such as tungsten and iridium, valuable in applications requiring high temperature resistance or structural integrity.
In conclusion, the strength of metallic bonds is primarily determined by the charge of the metal ions and their ionic radius. These factors vary systematically across the periodic table, leading to predictable trends in metallic bond strength and related properties. By understanding how these factors change across periods and down groups, we can better explain and predict the behavior of metals in various applications, from everyday objects to advanced technological materials.
Comparison of Metallic Bonding with Other Bond Types
Metallic bonding, ionic bonding, and covalent bonding are three fundamental types of chemical bonds that play crucial roles in determining the properties of various materials. While each type of bond has its unique characteristics, metallic bonding stands out due to its distinct features and the properties it imparts to metals.
Metallic bonding occurs between metal atoms and is characterized by the presence of delocalized electrons. In this type of bonding, metal atoms arrange themselves in a lattice structure, with their outer electrons forming a "sea" of freely moving electrons. This electron sea is responsible for many of the unique properties of metals, such as their high electrical conductivity and thermal conductivity.
In contrast, ionic bonding involves the transfer of electrons between atoms, typically from a metal to a non-metal. This results in the formation of oppositely charged ions that are held together by electrostatic forces. Sodium chloride (table salt) is a classic example of an ionic compound. Covalent bonding, on the other hand, involves the sharing of electrons between atoms, usually between non-metal elements. Water (H2O) and carbon dioxide (CO2) are common examples of covalently bonded molecules.
The unique features of metallic bonds set them apart from ionic and covalent bonds. The delocalized electrons in metallic bonds allow for the easy movement of charge, explaining why metals are excellent conductors of electricity and heat. This is in stark contrast to ionic compounds, which typically conduct electricity only when molten or in solution, and covalent compounds, which are often poor conductors.
Another distinguishing feature of metallic bonding is the malleability and ductility it imparts to metals. The ability of metal atoms to slide past one another while maintaining the overall bonding structure allows metals to be shaped and drawn into wires without breaking. Ionic compounds, being brittle, and covalent compounds, often existing as discrete molecules, do not exhibit these properties.
The formation of metallic bonds is favored by elements with relatively few valence electrons and low electronegativity. These elements, typically found on the left side of the periodic table, readily give up their outer electrons to form the electron sea characteristic of metallic bonding. Elemental iron, for instance, exhibits strong metallic bonding, contributing to its strength and widespread use in construction and manufacturing.
In contrast, elements that form ionic bonds are usually those with a large difference in electronegativity, such as metals and non-metals. Sodium and chlorine, for example, form ionic bonds in sodium chloride due to the significant difference in their electron-attracting abilities. Covalent bonds, however, typically form between non-metal elements with similar electronegativities, allowing them to share electrons rather than transfer them completely.
The physical properties of substances also reflect the type of bonding present. Metals, due to their metallic bonding, generally have high melting and boiling points, good electrical conductivity and thermal conductivity, and a characteristic luster. Ionic compounds often have high melting points but are brittle and conduct electricity only when molten or in solution. Covalent compounds can vary widely in their properties, from gases like oxygen to network solids like diamond, depending on the strength and arrangement of their bonds.
Understanding these different types of bonding is crucial in materials science and chemistry. The type of bonding present in a substance determines its physical and chemical properties, which in turn dictate its potential applications. For instance, the metallic bonding in copper makes it an ideal material for electrical wiring, while the covalent bonding in plastics allows for their versatility in manufacturing.
In conclusion, while metallic, ionic, and covalent bonding all involve the interaction of electrons between atoms, they result in substances with vastly different properties. Metallic bonding, with its unique electron sea model, stands out for the distinctive properties it imparts to metals, making them indispensable in numerous technological and industrial applications.
Applications and Importance of Metallic Bonding
Metallic bonding, a fundamental concept in chemistry and materials science, plays a crucial role in various fields and industries. Understanding the practical applications and importance of metallic bonding is essential for advancements in metallurgy, electronics, and material science. This knowledge enables the development of new materials and technologies that shape our modern world.
In metallurgy, metallic bonding is the cornerstone of alloy design and production. The unique properties of metals, such as malleability, ductility, and conductivity, are direct results of metallic bonding. Metallurgists leverage this understanding to create stronger, lighter, and more corrosion-resistant alloys for use in construction, automotive, and aerospace industries. For example, the development of high-strength steel for skyscrapers and bridges relies on manipulating metallic bonds to enhance structural integrity.
The electronics industry heavily depends on metallic bonding principles. Conductors, semiconductors, and superconductors all owe their electrical properties to the behavior of electrons in metallic bonds. The design of integrated circuits, microprocessors, and other electronic components is based on a deep understanding of how electrons move through metallic structures. This knowledge has led to the miniaturization of electronic devices and the continuous improvement in computing power.
In material science, metallic bonding is a key factor in developing advanced materials with tailored properties. Researchers exploit the characteristics of metallic bonds to create materials with specific thermal, magnetic, or optical properties. For instance, shape memory alloys, which can return to their original shape after deformation, are a result of manipulating metallic bonds at the atomic level. These materials find applications in medical devices, aerospace, and robotics.
The automotive industry benefits significantly from metallic bonding knowledge. Lightweight alloys developed through an understanding of metallic bonding help improve fuel efficiency and reduce emissions. Additionally, the development of catalytic converters, which rely on the unique properties of platinum and palladium, is rooted in metallic bonding principles.
In everyday life, we encounter numerous applications of metallic bonding. From the aluminum cans we use for beverages to the copper wires that power our homes, metallic bonding is omnipresent. The durability of kitchen utensils, the effectiveness of heat sinks in computers, and the reliability of electrical connections all stem from the properties conferred by metallic bonds.
The energy sector also benefits from metallic bonding applications. The development of more efficient solar panels, wind turbines, and batteries for energy storage relies on optimizing metallic structures. For example, improving the efficiency of photovoltaic cells often involves manipulating the metallic contacts to enhance electron flow.
In the field of nanotechnology, understanding metallic bonding at the atomic scale has led to breakthroughs in creating nanoparticles and nanostructures with unique properties. These have applications in medicine, environmental remediation, and advanced sensing technologies.
The importance of metallic bonding extends to the realm of 3D printing and additive manufacturing. By understanding how metals bond and behave at different temperatures, engineers can design better 3D printing processes for metals, opening up new possibilities in rapid prototyping and custom manufacturing.
In conclusion, the practical applications and importance of metallic bonding are vast and far-reaching. From the cars we drive to the smartphones we use, metallic bonding underpins countless technologies and materials that define modern life. As research in this field continues, we can expect even more innovative applications that will shape the future of technology and industry. The ongoing study of metallic bonding remains crucial for addressing global challenges in energy, transportation, and sustainable development.
Conclusion
Metallic bonding is a crucial concept in chemistry, characterized by the sharing of delocalized electrons among positively charged metal ions. This unique bonding type explains the distinctive properties of metals, such as high electrical conductivity and malleability. The introduction video provides an essential foundation for understanding metallic bonding, illustrating how the "sea of electrons" model contributes to these properties. To deepen your knowledge, explore further aspects of metallic bonding, including its role in alloy formation and its applications in materials science. Understanding metallic bonding is vital for comprehending the behavior of metals in various chemical bonding types. As you continue your journey in chemistry, consider how metallic bonding relates to other types of chemical bonding, such as ionic and covalent bonds. This interconnected knowledge will enhance your overall understanding of chemical structures and reactions. We encourage you to pursue additional resources and experiments to reinforce your grasp of metallic bonding and its significance in the world of chemistry and materials science.
Metallic Bonding: An In-Depth Guide
What is metallic bonding? Features of metallic bonding.
Step 1: Introduction to Metallic Bonding
In this lesson, we will explore a third type of bonding known as metallic bonding. Having already covered ionic and covalent bonding, metallic bonding presents an alternative type of bonding that is primarily found in pure metals and elemental metals. This type of bonding is crucial for understanding the properties and behaviors of metals.
Step 2: Understanding the Basics
Metallic bonding occurs in pure elemental metals such as iron, copper, and nickel. Unlike ionic or covalent bonding, which involve the attraction between positive and negative ions or the sharing of electron pairs, metallic bonding involves a different mechanism. In metallic bonding, metal atoms release some of their electrons to form a 'sea' of delocalized electrons. These electrons are not bound to any specific atom and can move freely throughout the metal lattice.
Step 3: The Structure of Metallic Bonding
The structure of metallic bonding can be visualized as a lattice of positive metal ions surrounded by a sea of delocalized electrons. The positive metal ions are arranged in a regular, repeating pattern, forming a lattice. The delocalized electrons move freely between these ions, creating a strong electrostatic attraction between the positively charged ions and the negatively charged electrons. This attraction holds the metal together.
Step 4: Features of Metallic Bonding
There are several key features of metallic bonding:
- Delocalized Electrons: The electrons in metallic bonding are not fixed in place. They can move freely throughout the metal, which allows metals to conduct electricity and heat efficiently.
- Positive Metal Ions: The metal atoms lose some of their electrons to form positive ions. These ions are arranged in a regular, repeating lattice structure.
- Electrostatic Attraction: The attraction between the positive metal ions and the sea of delocalized electrons creates a strong bond that holds the metal together.
- Lattice Structure: The positive metal ions form a regular, repeating lattice structure. This structure is not as rigid as the lattice found in ionic or covalent compounds, allowing metals to be malleable and ductile.
Step 5: Properties of Metals Explained by Metallic Bonding
Metallic bonding explains many of the properties of metals:
- Electrical Conductivity: The free movement of delocalized electrons allows metals to conduct electricity efficiently.
- Thermal Conductivity: The delocalized electrons can also transfer heat energy quickly, making metals good conductors of heat.
- Malleability and Ductility: The lattice structure of positive metal ions allows metals to be hammered into thin sheets (malleability) or drawn into wires (ductility) without breaking.
- Luster: The presence of delocalized electrons on the surface of metals reflects light, giving metals their characteristic shiny appearance.
Step 6: Occurrence of Metallic Bonding
Metallic bonding occurs in pure metal samples and alloys. Alloys are mixtures of metals that retain the properties of metallic bonding. It is important to note that metallic bonding does not occur in ionic compounds such as sodium chloride (NaCl) or in metal oxides and chlorides. These compounds exhibit ionic bonding instead.
Step 7: Conclusion
In summary, metallic bonding is a unique type of bonding found in pure metals and alloys. It involves a lattice of positive metal ions surrounded by a sea of delocalized electrons. This bonding mechanism explains many of the characteristic properties of metals, such as electrical and thermal conductivity, malleability, ductility, and luster. Understanding metallic bonding is essential for comprehending the behavior and properties of metals in various applications.
FAQs
Here are some frequently asked questions about metallic bonding:
-
What is a metallic bond in simple terms?
A metallic bond is a type of chemical bond that occurs between metal atoms. In this bond, the outer electrons of metal atoms are shared in a "sea" of delocalized electrons, while the positively charged metal ions form a lattice structure. This arrangement allows for the unique properties of metals, such as high electrical conductivity and malleability.
-
What is the difference between metallic and covalent bonding?
Metallic bonding involves the sharing of electrons among many metal atoms, creating a sea of delocalized electrons. Covalent bonding, on the other hand, involves the sharing of electrons between specific atoms to form discrete molecules. Metallic bonds result in properties like conductivity and malleability, while covalent bonds often form gases, liquids, or network solids with different properties.
-
How do metallic bonds stay together?
Metallic bonds are held together by the electrostatic attraction between the positively charged metal ions and the negatively charged sea of delocalized electrons. This strong attraction keeps the metal atoms in a cohesive structure, allowing for the characteristic properties of metals.
-
What are some examples of metallic bonds?
Examples of metallic bonds can be found in pure metals like copper, silver, gold, iron, and aluminum. Alloys, such as steel (iron and carbon) and brass (copper and zinc), also exhibit metallic bonding. These materials showcase properties like electrical conductivity, thermal conductivity, and malleability due to their metallic bond structure.
-
Do metallic bonds have high melting points?
Generally, yes. Metallic bonds often result in high melting points due to the strong attraction between the positive metal ions and the sea of electrons. However, the melting point can vary depending on the specific metal. Some metals, like tungsten, have extremely high melting points (3,422°C), while others, like mercury, have low melting points (-38.83°C). The strength of the metallic bond, influenced by factors such as the number of valence electrons and atomic size, determines the melting point.
Prerequisite Topics for Understanding Metallic Bonding
To fully grasp the concept of metallic bonding, it's crucial to have a solid foundation in several key areas of chemistry. Understanding these prerequisite topics will significantly enhance your ability to comprehend the unique properties and behaviors of metals in chemical interactions.
One of the most important concepts to master is electrical conductivity. Metals are known for their high electrical conductivity, which is a direct result of their bonding structure. This property is fundamental to metallic bonding, as it explains how electrons can move freely within the metal lattice, contributing to the characteristic "sea of electrons" model.
Another critical prerequisite is understanding ionic and covalent bonding. While metallic bonding is distinct from these types, comparing and contrasting it with ionic and covalent bonding helps in appreciating the unique nature of metallic bonds. Unlike covalent bonding, where electrons are shared between specific atoms, metallic bonding involves a more delocalized sharing of electrons across the entire structure.
Familiarity with the history and development of the periodic table is also invaluable. The periodic table trends play a crucial role in predicting and explaining metallic properties. Understanding how elements are arranged and how their properties change across periods and groups provides insight into why certain elements form metallic bonds while others don't.
The concept of electrical conductivity in metals is directly linked to their bonding structure. In metallic bonding, the outer electrons of metal atoms are loosely held and can move freely throughout the metal's structure. This "sea of electrons" is what gives metals their high electrical conductivity. By understanding this prerequisite, students can better grasp why metals are excellent conductors and how this property relates to their bonding.
Knowledge of ionic and covalent bonding serves as a comparative basis for understanding metallic bonding. While ionic bonds involve the transfer of electrons and covalent bonds involve sharing electrons between specific atoms, metallic bonding is characterized by a sharing of electrons across the entire metal structure. This understanding helps in differentiating the unique properties of metals from those of ionic and covalent compounds.
Lastly, the periodic table trends are essential in predicting metallic behavior. Elements on the left side of the periodic table, particularly in groups 1-3, are more likely to form metallic bonds. Understanding these trends helps in predicting which elements will exhibit metallic properties and how these properties might vary across the periodic table.
By mastering these prerequisite topics, students will be well-equipped to delve into the complexities of metallic bonding, understanding not just the what, but also the why behind the unique properties and behaviors of metals in chemical interactions.
In this lesson, we will learn:
- To describe the features of metallic bonding and the structure of pure metals.
- How to explain the properties of metals using metallic bonding theory.
- How to explain the trends in properties of metals using metallic bonding theory.
Notes:
- We saw earlier that ionic and covalent bonding are bonding types that hold compounds and small molecules together, but in elemental metal samples (pure metals, not metal compounds) there is a third type of bonding called metallic bonding.
- In elemental metals (that means pure iron metal, not iron compounds), metallic bonding creates a structure with the following features:
- There is a lattice of positively charged metal ions.
- Between these positive ions, there is a sea of negative delocalised electrons. These are the electrons that the metal atoms (that are now ions!) had lost, so they could gain a full outer shell.
- Metallic bonding, occurs in samples of metal-only atoms, including pure metallic samples, and explains the properties of pure metals that we observe.
- Metallic bonding occurs in PURE METAL SAMPLES. It occurs in alloys too, which are mixtures of different metals we will look at next lesson.
- Metallic bonding does NOT occur in metal compounds with non-metal atoms. That is ionic bonding which we learned in C11.4.2: Ionic and covalent bonding
- As with any bonding theory, we use our ideas of metallic bonding to help explain the properties that we see when we study metals. Metallic bonding explains the properties of metals in the following ways:
- There is a strong electrostatic attractive force between the metal ions and the delocalised electrons. It takes a lot of energy to overcome this force and pull the positive ions apart from the delocalised electrons. This is why most metals have a high melting point.
- The sea of delocalised electrons is fluid. This means the metal ions can move amongst and around each other because they arent rigidly stuck in one place in the lattice. This is why many pure metals are both malleable and ductile:
- A material that is malleable can be bent and re-shaped when it is heated up. This is how blacksmithing works (how swords and iron tools are made), as hot metal is hammered into different shapes before it hardens as it cools.
- A material that is ductile can be bent and drawn into thin wires. Copper is very ductile and most electrical wires are made from it.
- The fluid sea of negatively charged delocalised electrons easily carry electric charge and heat energy throughout the lattice.
- This explains why metals are good conductors of both electricity and heat.
- There are trends in properties of metals, like their melting point. The trends are caused by different metallic bonding strength which is caused by two main factors:
- The charge of the metal ion in the lattice. For example, compare group 1 metals that have a 1+ ion charge and group 2 metals with ions of 2+ charge. If you compare a group 1 and group 2 metal in a period like Na and Mg, the group 2 metal will have a higher melting point because of the greater charge difference.
In short, 2+ attracting 2- is a stronger force than 1+ attracting 1-. - The ionic radius of the ion. In Periodic trends: Atomic radius, we saw that the ionic radius gets larger going down a group in the periodic table.
This means the nucleus (where the positive charge is) is further away from the delocalised electrons it is attracted to, so a larger ionic radius makes a weaker metallic bond. You can see this in the melting and boiling points of metal elements decreasing down the group column. - Metallic structure and bonding is not only seen in pure metals, but also in mixtures of different metals combined ‐ these are called alloys. Alloys are made to obtain unique or more precise properties of two or more metal elements. We will look at alloys in our next lesson, Alloys.
See the diagram below but remember that the electrons are freely moving, thats why they look a bit disorganised.
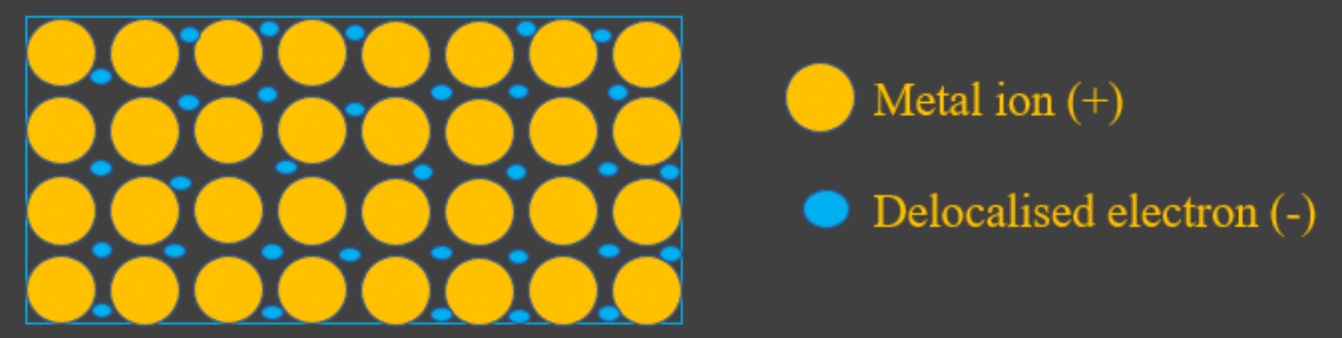
remaining today
remaining today