In this lesson, we will learn:
- Waves superposition
- Waves interference
- Standing waves
Notes:
Superposition (When two or more waves travelling though one another)
- As waves pass through each there is no change in:
Amplitude, direction, speed, frequency, wavelength - The displacement at the point where they meet is the sum of the displacements.
- When pulses pass through one another superposition takes place.
Interference
- When pulse meet at a point at the same time interference occurs.
- Interference is the result of the superposition of two or more waves (the point where they meet).
- Constructive interference: waves displacement are in the same direction, displacements add up, maximum displacement (ANTINODE)
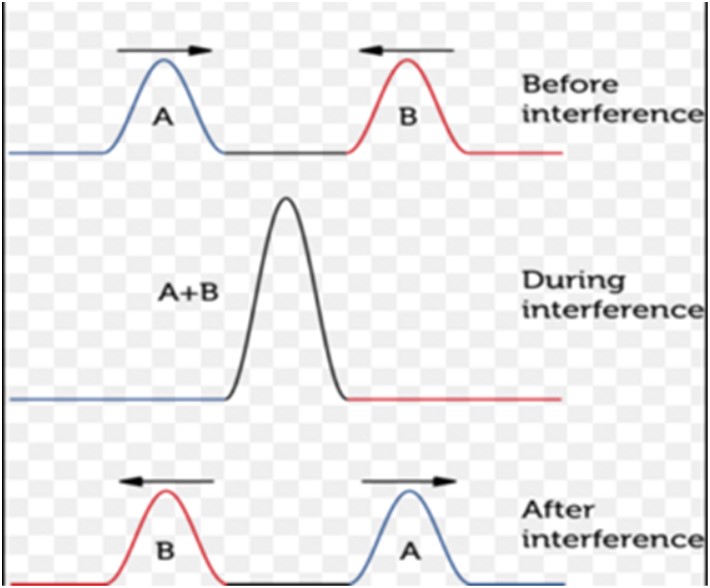
- Destructive interference: waves displacement are in the opposite direction, displacements cancel out, zero displacement (NODES)
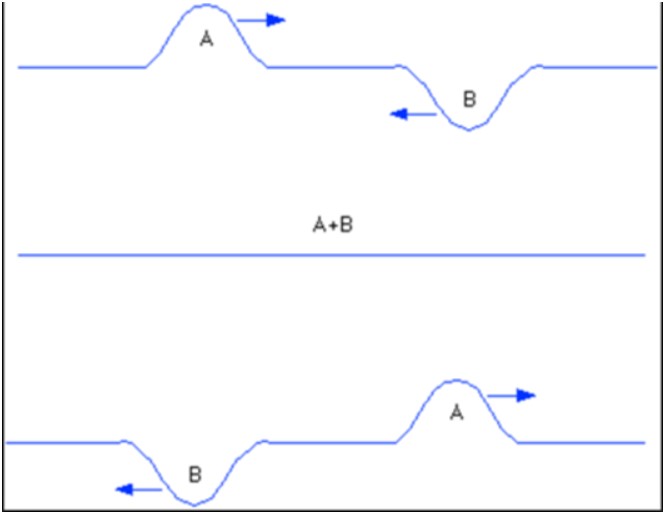
Standing waves
- When a string is plugged pulses travel back and forth, reflecting from the ends producing a complex of superposition called standing waves. Superimposed waves can produce standing waves.
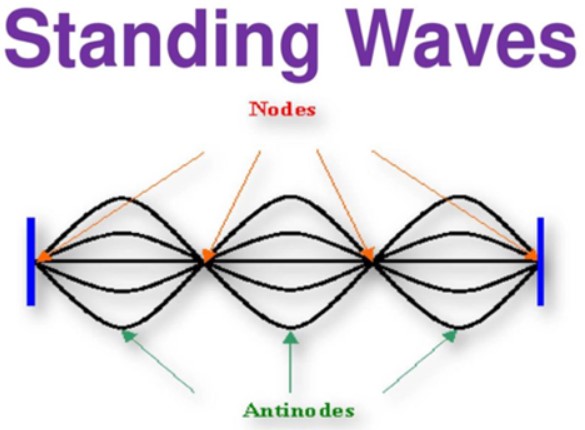