In this lesson, we will learn:
- What is light?
- Source of light
- Light is the visible part of electromagnetic waves
- Light range frequencies
- Visible light colors and wavelengths
- Ray model of light
- Solving problems involving the speed of light
- Solving illumination problems
Notes:
Introduction to Light Fundamentals
- Light is emitted and reflected from objects around us
- Light is a small visible part of electromagnetic waves.
- Light travel along straight line in vacuum or other uniform medium
- The nature of light is explained by two models; particle model and wave model
- Light frequency changes upon moving along the electromagnetic spectrum
- Light is the range of frequencies of electromagnetic waves
- Light waves have wavelength about 400nm to 700nm
- Shortest wavelengths are seen as violet light
- Longest wavelengths are seen as red light
- Knowing that light travels along a straight line in vacuum or any uniform medium leads us to the ray model of light.
- A ray of light is a straight line representing the path of a narrow beam of light.
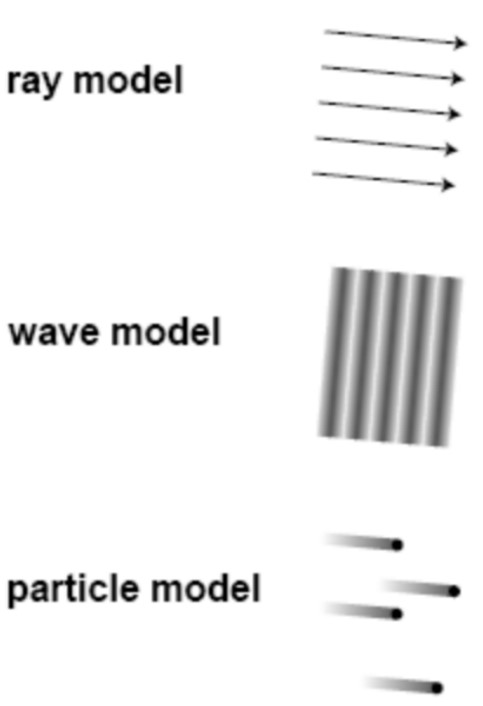
Speed of Light
- Speed of light is represented by letter
- Speed of light is constant in the air and is 3.00×108m/s
- Recall the equation to find the speed of waves, we can modify the equation in terms of speed of light
Luminous body Vs. Illuminated body
- Luminous body emits light waves (sun, lamp)
- Illuminated body reflects waves produced by an outside source (moon, bridge)
- Luminous flux, is the rate at which light is emitted from a source
- Unit of luminous flux is the lumen
- The mount of illumination of a surface called illuminance,
lumens per square meter OR lux, lx
luminous flux of the source
distance between the surface and the source