In this lesson, we will learn:
- To explain the bonding in simple molecules using atomic orbital hybridization.
- To correctly draw sp3, sp2 and sp hybridized atomic orbitals.
- To explain how atomic orbital hybridization is consistent with the observed properties of carbon-carbon bonds.
- In the lesson Atomic orbitals and energy levels, we learned that we use atomic orbitals (AOs) to explain bonding. We write electron configurations, like 1s2 2s2 2p2, to show electrons in different atomic orbitals that create molecules out of separate atoms by taking part in bonding.
But atomic orbitals on their own don’t accurately reflect the bond energies and bond lengths in compounds. For example, carbon’s electron configuration is [1s2] 2s2 2p2. The two 2s electrons are paired up and two p electrons are unpaired in the higher energy 2p subshell. So it has four valence electrons and two are in a higher energy state than the other two.
Does carbon actually make two bonds with a lone pair, like an analogue of NH3? Let’s look at two carbon compounds: - Methylene (:CH2) is a type of compound called a carbene. Carbenes have two electrons on carbon that are not shared; the other two are making covalent bonds. However, methylene is very reactive and unstable – most simple carbenes are.
- In methane (CH4),carbon is bonded to four hydrogens and the bond angles and strengths are all equal. Methane is a stable compound that is easily stored at standard conditions.
- Atomic orbital hybridization explains why in methane (CH4), carbon’s four valence electrons do not bond in this 2s2 2p2 ground state. If they did, there would be marked differences in bond energy. The four n=2 atomic orbitals (AOs) mix together to create four equivalent hybrid orbitals that are equal in energy.
- This is done by a 2s electron being excited to a slightly higher 2p orbital, the one that is currently empty.
This results in four singly occupied orbitals; three 2p orbitals equal in energy and one 2s orbital slightly lower in energy. Now carbon can form four single bonds. - These four orbitals mix together to create four hybrid orbitals of equal energy.
In methane the one 2s orbital and three 2p orbitals create four sp3 hybrid orbitals. The character of the hybrids is related to the AOs that made them, so they are 25% ‘s character’ and 75% ‘p character’. - These sp3 hybrid orbitals can combine by overlapping with orbitals in other atoms (like the 1s AO of hydrogen) and form a sigma bond. Sigma bonds are formed by head-on orbital overlap.
- Hybridization can explain double bonds too, such as in ethene (C2H4). As with any scientific theory, hybridization must be able to explain the experimental evidence. Bond enthalpy data clearly shows C=C double bonds are less than twice as strong as two C-C single bonds. Something about the double bond orbital overlap is not as attractive in nature as the single bond overlap.
- Ethene (C2H4) has its two carbon atoms bonded to three other atoms; two hydrogens each and the other carbon atom in the molecule. After a 2s electron is excited up to the empty 2p orbital, only the 2s and TWO 2p AOs need to be hybridized, not all three like in CH4. They are therefore called sp2 hybrid orbitals. They are one-third s character and two-thirds p character.
- The last unhybridized 2p orbital containing one electron, which both carbon atoms have, will form a pi bond. Pi bonds are formed by planar orbital overlap, i.e. orbitals perpendicular to the positive nuclei, not in between them like the sigma bond. This out-of-plane pi interaction is further from the attractive nuclei and is therefore less strong than the sigma bond. This is why C=C bonds are not twice as strong as C-C single bonds.
- Hybridization can be used to show the bonding for a carbon triple bond, too. Carbon in ethyne (the simplest alkyne) is sp hybridized . How many orbitals do you think will be hybridized here?
- Ethyne has only two atoms bonded to each carbon atom – it only needs to hybridize for a C-C and C-H sigma bond, taking the 2s orbital and one 2p orbital, hence it the label sp and 50:50 s and p character. The two perpendicular unhybridized p orbitals form the two pi bonds between the two carbon atoms to make the triple bond overall. See the diagram below.
- Equally as we saw with ethene, ethyne has a carbon-carbon triple bond, which is not three times as strong as a C-C single bond because it is made of two pi bonds which are weaker than sigma bonds.
- The type of hybridization a carbon atom has with its orbitals is easily found by the number of atoms the carbon center is bonded to:
- Orbital hybridization is an extension of valence bond theory , which helps us explain the bonding and geometry in many compounds. It has a few basic principles:
- Electrons exist in atomic orbitals or hybrid atomic orbitals which are localized to their principle atoms.
- Electrons in atomic or hybrid orbitals repel each other so will maximise space between them around a central atom (similar to how VSEPR finds its bond angles).
- Chemical bonds are formed by the overlap of separate atomic or hybrid orbitals, where sigma bonds are created by constructive head-on overlap of orbitals, and pi bonds are created by constructive planar overlap of orbitals.
So how does carbon in CH4 make four bonds? How are the C-H bonds all equal energy and length? One current theory of bonding is that atomic orbitals can mix together or hybridize to become hybrid orbitals that are equal in energy. We call this idea atomic orbital hybridization. Like a hybrid animal or car, hybrid atomic orbitals are mixes of different atomic orbitals that show the characteristics of both.
Like in VSEPR, the electrons in different orbitals repel one another so they will find maximum spacing around the atom, which for tetrahedral carbon is 109.5°. This explains the observation of equal C-H bond angles and bond strength in methane. These hybrids can be drawn as a mix of s and p orbitals, where they do still look similar to the p orbitals they have 75% of the character of, with the s character shown as one lobe of the p orbitals enlarged, the other shrunk (due to destructive/constructive overlap). See below for a diagram:

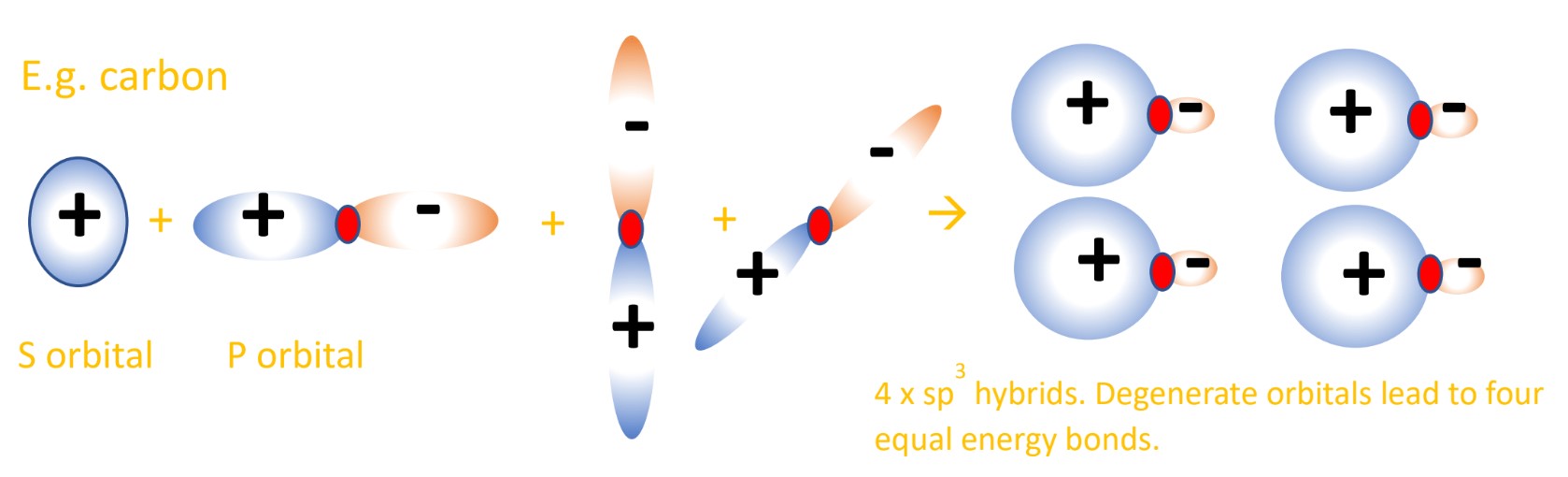
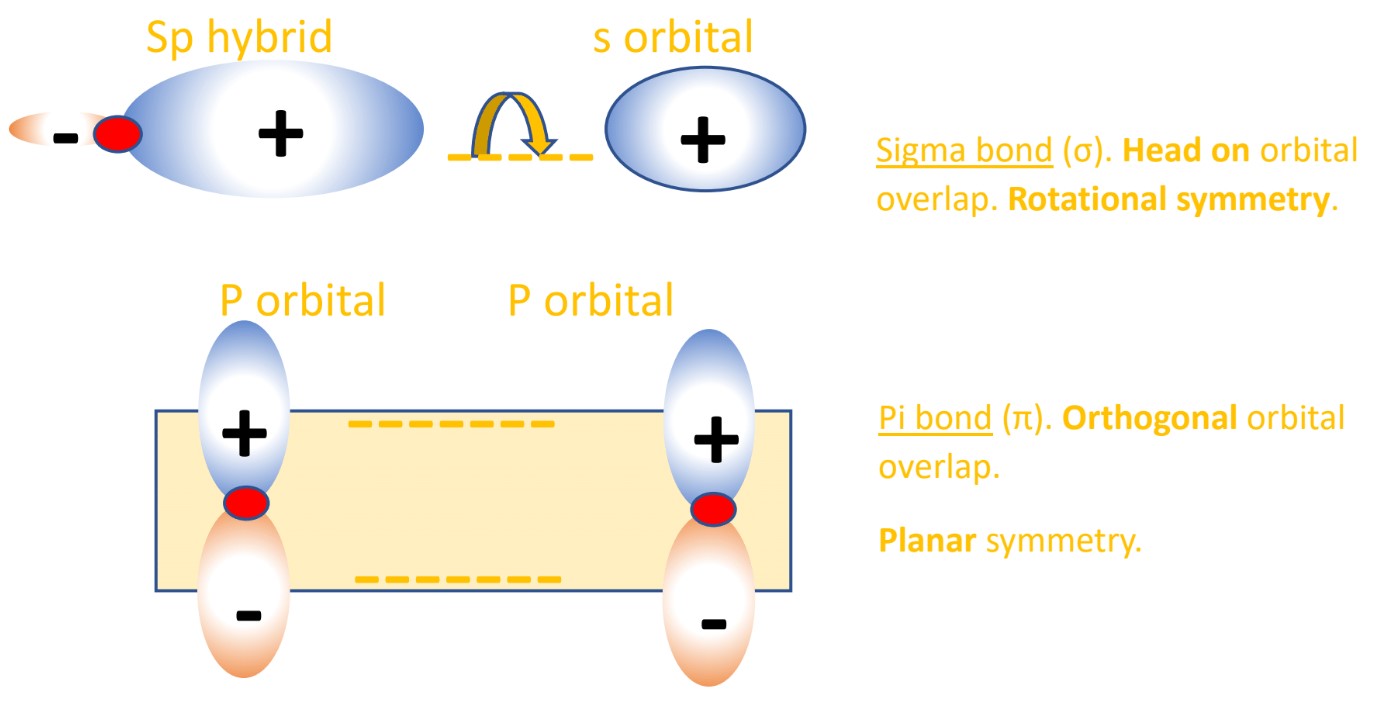
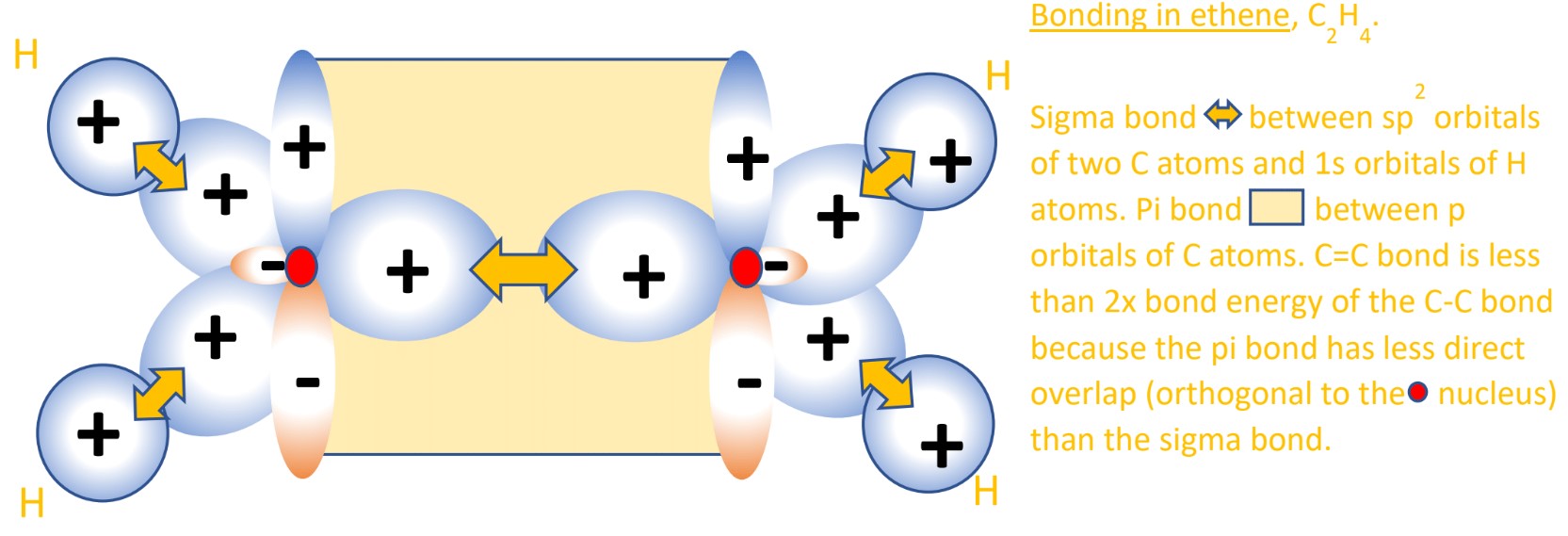
|
|
|
|
|
|
|
|
|
|
|
|
|
|
|
|
While this does accurately predict the properties of many compounds, it falls short in some areas.
Another theory of chemical bonding is known as molecular orbital theory, which also accurately predicts the properties of many compounds, succeeding in places where valence bond theory does not. This is our focus for the next lesson.