- The changes made to the Periodic Table since Mendeleev's major contribution.
- The basic structure and layout of the modern Periodic Table.
- The precise grouping and arrangement of the Periodic Table.
Notes:
- As seen in the lesson on History and Development of the Periodic Table, one of the main reasons the development of the Periodic Table was initially slow is because data quality was often poor. Inaccurate measurements meant organizing elements correctly was hard.
- As improvements meant more and better data could be used, some changes were made to Mendeleev's Periodic Table. One big change was in ordering the table by atomic number (proton number) rather than by atomic mass. This solved the problem of isotopes; when arranged by atomic mass, some elements look like they are in the wrong place in the table (e.g. K has a lower atomic mass than Ar, even though K has one more proton). The current periodic table, then, obeys the Periodic Law: The properties of the elements repeat periodically when ordered according to their atomic numbers.
- The basic layout of the current periodic table has:
- Columns known as groups, the elements inside each of which having similar properties.
- Rows known as periods. The elements in a given period have the same number of electron shells.
- The current periodic table is arranged in a way that separates metals and non-metals:
- Metals are found on the left hand side of the periodic table.
- Non-metals are found on the right hand side of the periodic table.
- Metalloids or semiconductors are found on the border between metals and non-metals. These elements have a mix of metal and non-metal properties.
- About 80% of the elements in the periodic table are metals. The 'border' between metals and non-metals begins with boron and runs diagonally down to between non-metal Astatine (At) and Polonium metal (Po).
- The current periodic table has its specific shape to clearly show the different "blocks" of elements, in terms of their electron subshells (see lesson Electronic structure: Subshells).
- The first two groups (columns) form the s-block, the taller section on the left. This contains the alkali metals (group 1) and the alkali earth metals (group 2).
- The next ten groups form the d-block, generally known as the transition metals. This is the central block where the table 'dips'.
- The next six groups, where the table rises up again, is the p-block. It contains the metalloids and non-metals, including the halogens and the noble gases.
- The 'island' normally shown alone at the bottom of the periodic table is the f-block. It is actually an insert, inside the lower part of the d-block. It contains two rows known as the lanthanides and actinides.
- The periodic table has its specific shape to indicate the electron sub-shells and the block which a given element falls into. This is based on experimental data; the energy cost of removing the first outer shell electron (the ionization energy) from a sample of a given element follows a consistent pattern across the period (see lesson Periodic Trends: Ionization energy). This pattern led chemists to develop the sub-shell theory where not all electrons in a shell are in the same state – some electrons were in s orbitals, others p, d or f orbitals. The outer electrons occupying these orbitals, as suggested by experimental data, is represented in the shape of the periodic table we use.
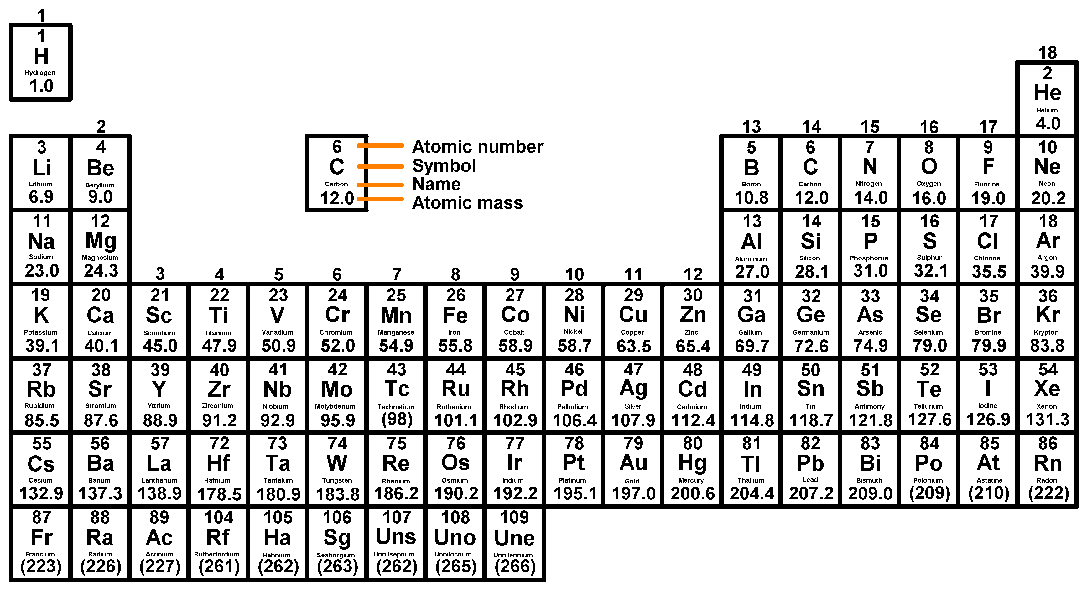