- The definition of an alkane, their general formula and the major types of them.
- The major properties of alkanes and differences to other hydrocarbons.
- How to name branched, unbranched and cyclic alkanes and draw their structural formula.
Notes:
- An alkane is a saturated hydrocarbon with only single bonds between carbon atoms.
- They are the simplest hydrocarbon compounds compounds that only contain carbon and hydrogen.
- Saturated means the compound contains online single bonds. Saturated compounds cannot hold any more hydrogen atoms: the maximum number of hydrogens is the CnH2n+2 that alkanes have.
- Alkanes are an example of a homologous series in organic chemistry.
- A homologous series is a set of compounds with the same general formula. Each compound in the set differs from the next by a -CH2- unit.
- Other examples of a homologous series are alkenes (general formula CnH2n), alkynes (CnH2n-2) and alcohols (CnH2n+2O).
- We saw in Organic chemistry introduction that carbon atoms can make strong bonds to other carbon atoms and form long chains in the process. You can have an alkane with a carbon chain length of three, five, twenty or fifty carbon atoms. The same is true of alcohols or alkenes.
- For example, the functional group in an alkene is the C=C double bond, which alkanes do not have. This C=C bond makes alkenes react in different ways to alkanes, which have only C-C single bonds. This is different to the O-H group in alcohols, which reacts differently to both.
- Alkanes have the general formula: CnH2n+2. This means that in an alkane, the number of hydrogen atoms is double the number of carbon atoms plus two. If a compound has a molecular formula that matches CnH2+2, it is an alkane.
- We get this general formula because every carbon atom in the chain is bonded to two other carbons in the chain and two hydrogen atoms each. The exception is the beginning and end carbons, which have three hydrogens each.
- Alkanes are unreactive compared to other hydrocarbons because they have no double or triple bonds – for them to react requires breaking a strong C-C bond which is difficult.
- Most alkanes are insoluble in water – they don't mix! They are more lipophilic (fat loving) than hydrophilic, so they are more easily dissolved in oils and fats instead of water.
- Hydrocarbons are flammable – methane, the simplest hydrocarbon, is also known as natural gas, and all of the shorter chain alkanes are volatile, flammable gases or liquids. Many of them are used as fuels!
- Alkanes will react with oxygen in a combustion reaction to produce carbon dioxide and water. For example, the reaction of methane and oxygen is given below:
- Straight chain alkanes are also called unbranched alkanes as there are no side chains. Each carbon is only bonded to two other carbon atoms in the middle of the chain, while the two chain ends are only bonded to one. They are named using the simple rules we saw in Organic chemistry introduction. See the table and examples below. Notice they all fit the alkane general formula: CnH2n+2.
- Branched alkanes or substituted alkanes contain carbon atoms in the middle of the chain bonded to carbon atoms that branch off of the main chain. This can create more than one possible 'chain lengths' – the longest chain is always considered the main chain, and the shorter ones are considered only branches. Naming branches uses the root words for the main chain, but ending in –yl to show it is a branch.
- Note one H atom is missing from the alkyl branch formulae. This because what would have been a C-H bond is instead a bond to the main carbon chain. See the table below:
- You must also add a number before this prefix, to show which carbon atom in the main chain the branch is found on. The numbering should always be the lowest possible.
- Using these rules we will look at two examples of hydrocarbons.
- In the first example, there are two branches of one carbon each – they are equal so it doesn't matter which you choose; one can be the main chain and the other the branch. So the longest chain is 5 carbons, with a single branch of 1 carbon. The 1 carbon branch is on the second carbon in the chain, because we always number using the lowest values possible (it could be on the 4th carbon if we counted the other way). So here have:
- A branch on the second carbon in the main chain: 2-
- A branch of 1 carbon length: methyl-
- A 5 carbon main chain alkane: pentane
- Combining these we have a compound named 2-methylpentane.
- In the second example, there are two end branches of two carbons each – again they are equal so we can pick one as the main chain and the other can be considered a branch. Again, number the branch off of the main chain with the lowest value possible, we will choose 3 instead of 4. We now have:
- A branch on the third carbon in the main chain: 3-
- A branch of 2 carbon atoms in length: ethyl-
- A 6 carbon main chain alkane: hexane
- Combining these, we have a compound named 3-ethylhexane.
- If branched alkanes have more than one type of branch, branches must be named in alphabetical order. For example, 'e' comes before 'm' in the alphabet, so ethyl chains should be named before methyl chains. See the example below:
- If you have an organic compound with more than one of the same type of branch or substituent, you need to say how many of these branches there are, and at which carbons in the chain they're found. This is done systematically:
- Two of the same substituents: di-
- Three of the same substituents: tri-
- Four: tetra-
- Five: penta-
- Six: hexa-
- Some hydrocarbons have the beginning and end of the chain bonded together, making a closed cyclic ring. These are unique chemical compounds with different properties to the open chain of the same carbon length. Because the ends of the chain are bonded together, cyclic alkanes have a different general formula to the rest of the alkanes. The general formula for cyclic alkanes is CH, like an alkene. To name cyclic compounds, the same basic rule of 'chain' length applies, with slight changes:
- Unbranched rings: Find the number of carbon atoms in the ring. This would be your original chain length. Then add 'cyclo-' in front. See the example:
- Rings with one branch or substituent: Start numbering from the ring carbon bonded to the branch. Because the ring has no 'start' or 'end', you can just pick the carbon bonded to the branch as number 1. Doing this is implied, we don't need to say 1-ethylcyclooctane, just ethylcyclooctane. See below:
- Rings with more than one branch or substituent: Start numbering in alphabetical order, and count from there following the lowest number rule. See the example below: ethyl comes before methyl in A-Z order, therefore it is named and numbered first. The rest of the ring is then numbered from here to give the lowest numbering possible (so 1-ethyl-3-methyl instead of going the other way around, which would give 1-ethyl-5-methyl).
- Notice that these branched alkanes and cycloalkanes can have the same molecular formula as some unbranched, straight chain alkanes and alkenes. These are examples of isomers – where the molecular formulae are the same but the structural formulae are different. This is very important as they are not the same chemical, they are unique and have different properties. This is another major reason why we use structural or skeletal formula when describing chemicals.
Alkanes, alkenes and alcohols are also families of compounds with different functional groups. The functional group is the most reactive part(s) of a molecule, so it usually identifies a substance in chemical reactions. It is the group that makes the compound function (react) the way it does.
This is an example of complete combustion where CO2 and H2O are produced, but there is also the possibility of incomplete combustion, where not enough oxygen is present to react the alkane. Below is the incomplete combustion of methane to produce carbon monoxide:
Incomplete combustion can produce carbon particulates (soot) when even less oxygen is available:
Which reaction occurs depends on how much oxygen is available. Look at the equations above; the complete combustion requires the most oxygen per mole of the alkane.
Incomplete combustion is undesirable for safety reasons, as the carbon monoxide released is highly toxic. It is also inefficient for engine performance, because incomplete combustion releases less energy overall than complete combustion does.
Length of main chain |
Root name |
Alkane name |
Molecular formula |
1 |
Meth- |
Methane |
CH4 |
2 |
Eth- |
Ethane |
C2H6 |
3 |
Prop- |
Propane |
C3H8 |
4 |
But- |
Butane |
C4H10 |
5 |
Pent- |
Pentane |
C5H12 |
6 |
Hex- |
Hexane |
C6H14 |
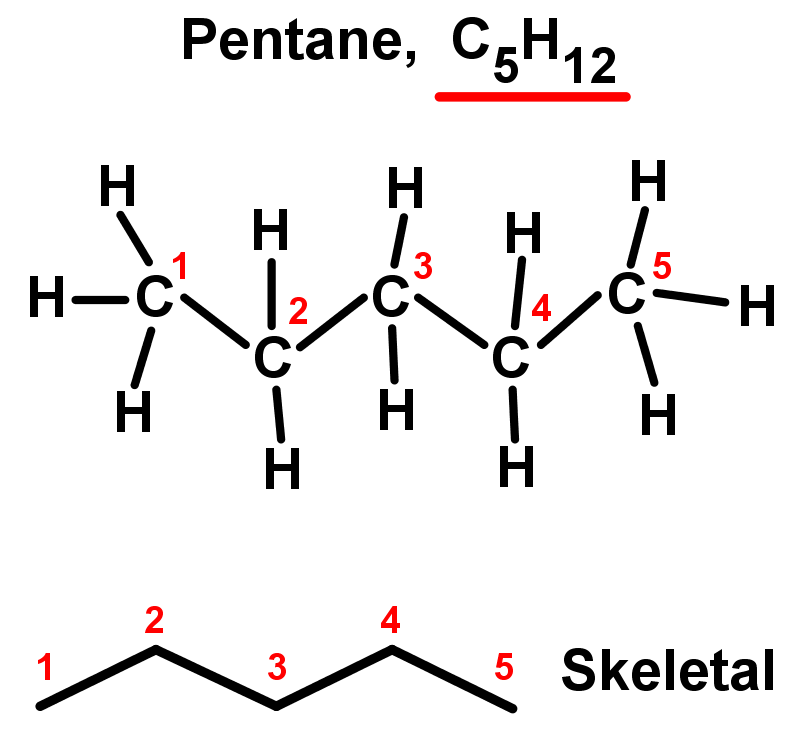
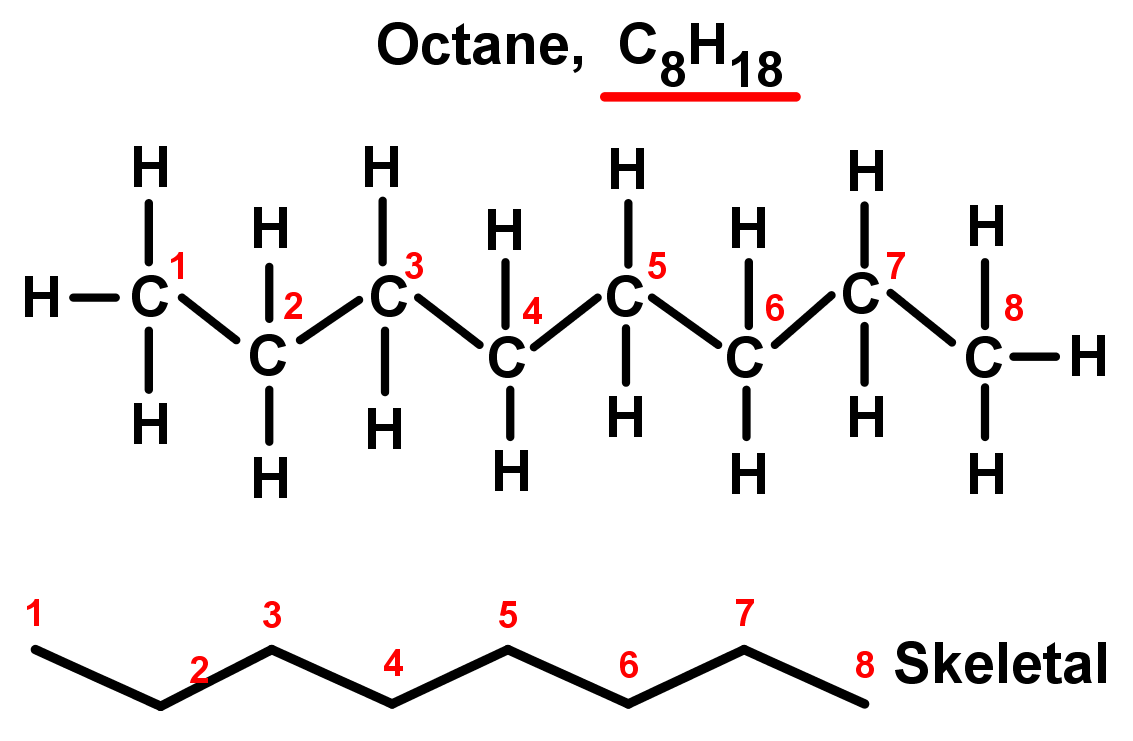
Chain length of branch |
Alkyl root |
Formula of alkyl branch |
1 |
Methyl- |
-CH3 |
2 |
Ethyl- |
-C2H5 |
3 |
Propyl- |
-C3H7 |
4 |
Butyl- |
-C4H9 |
5 |
Pentyl- |
-C5H11 |
6 |
Hexyl- |
-C6H13 |
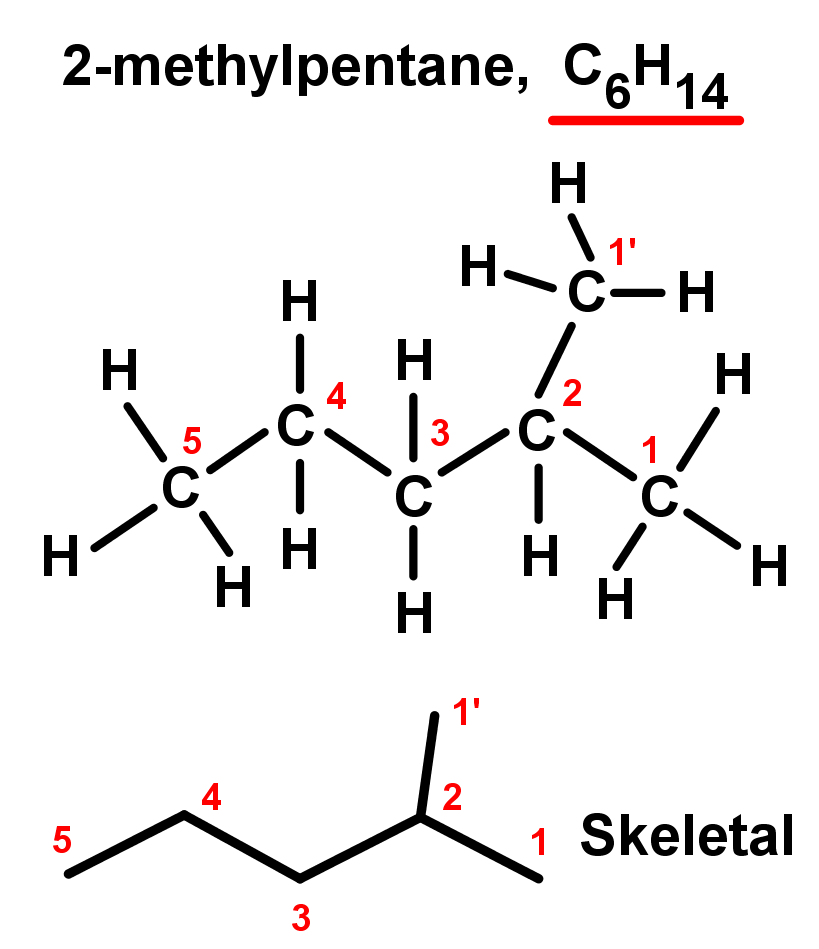
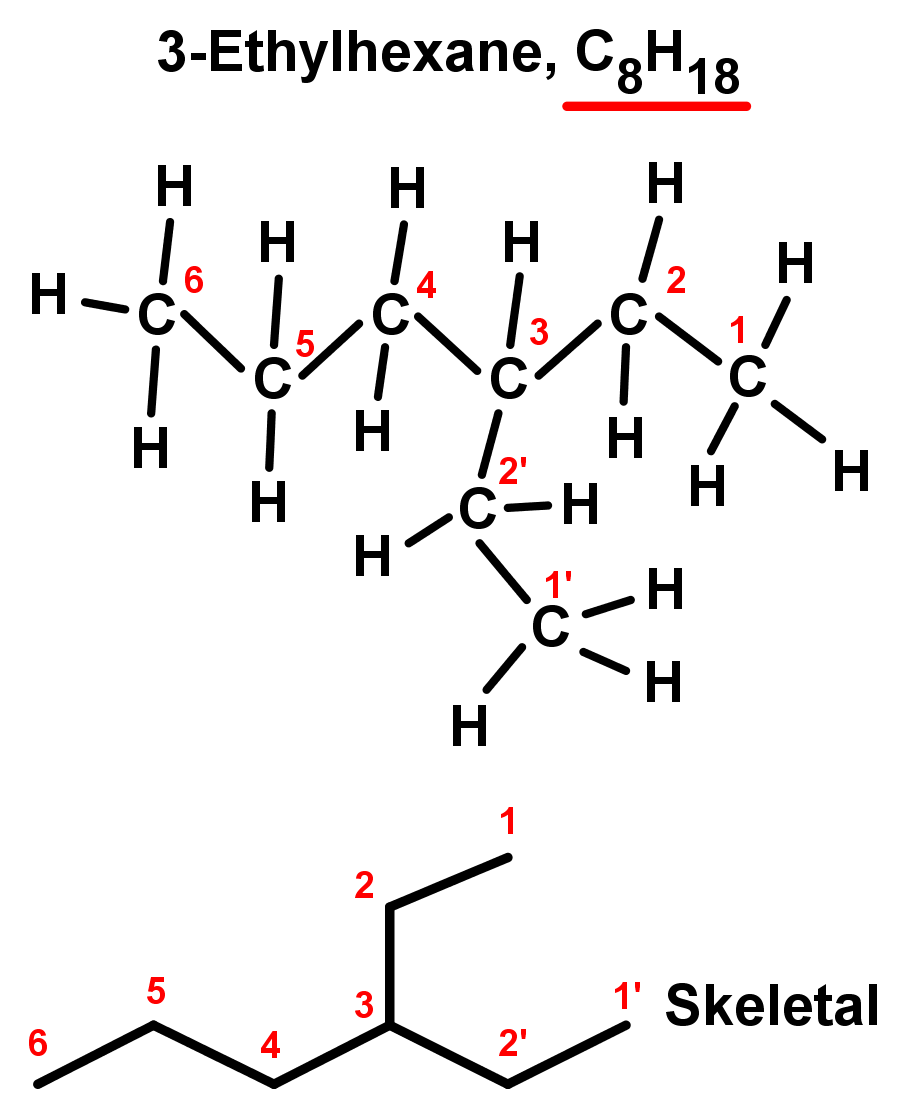
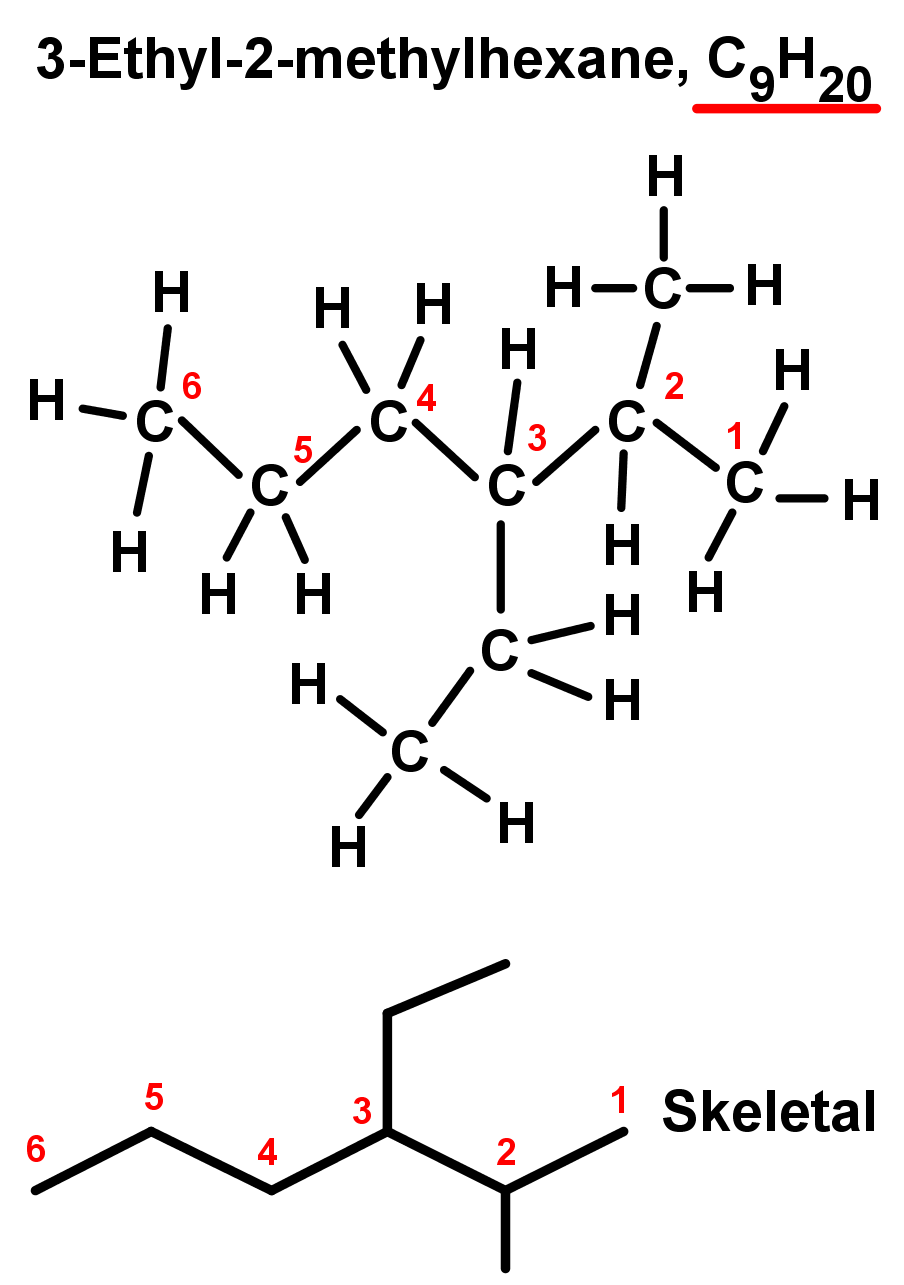
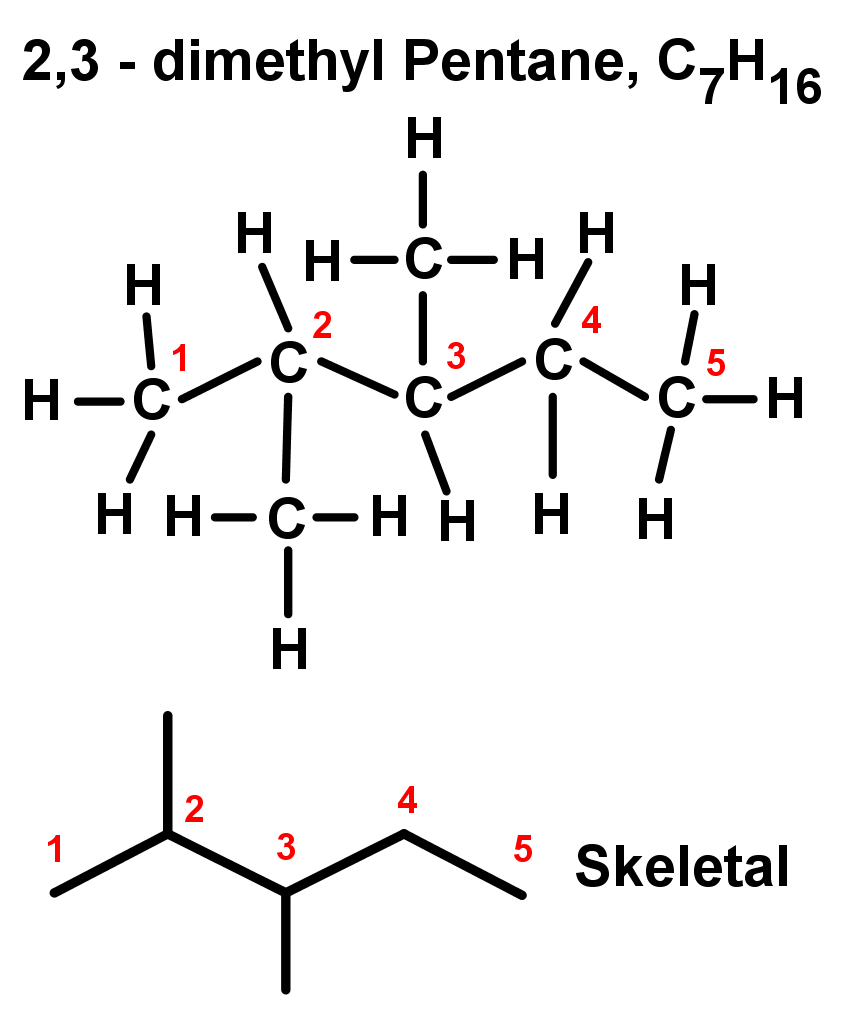
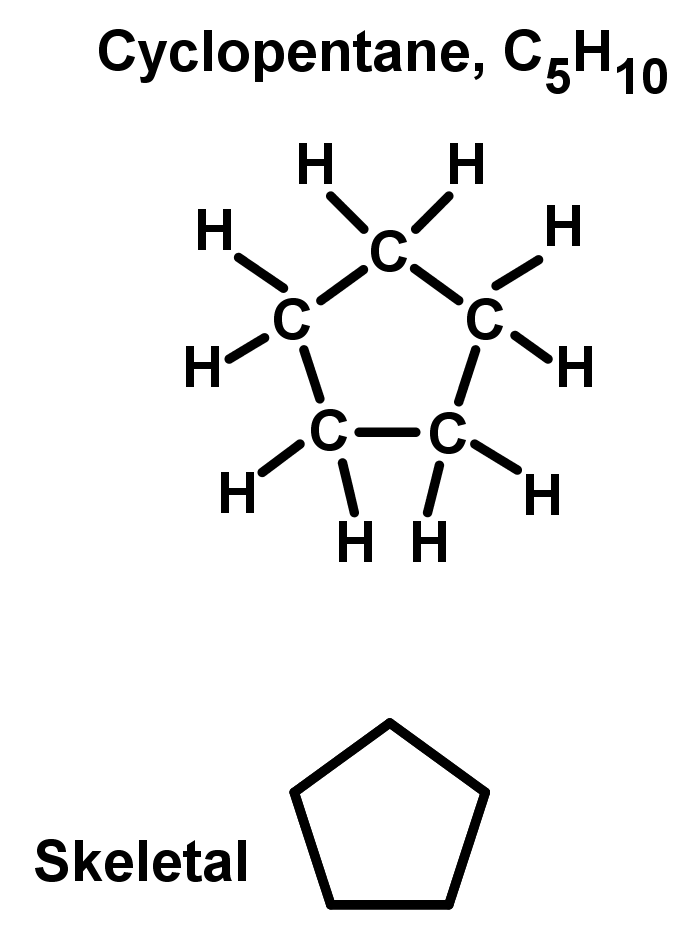
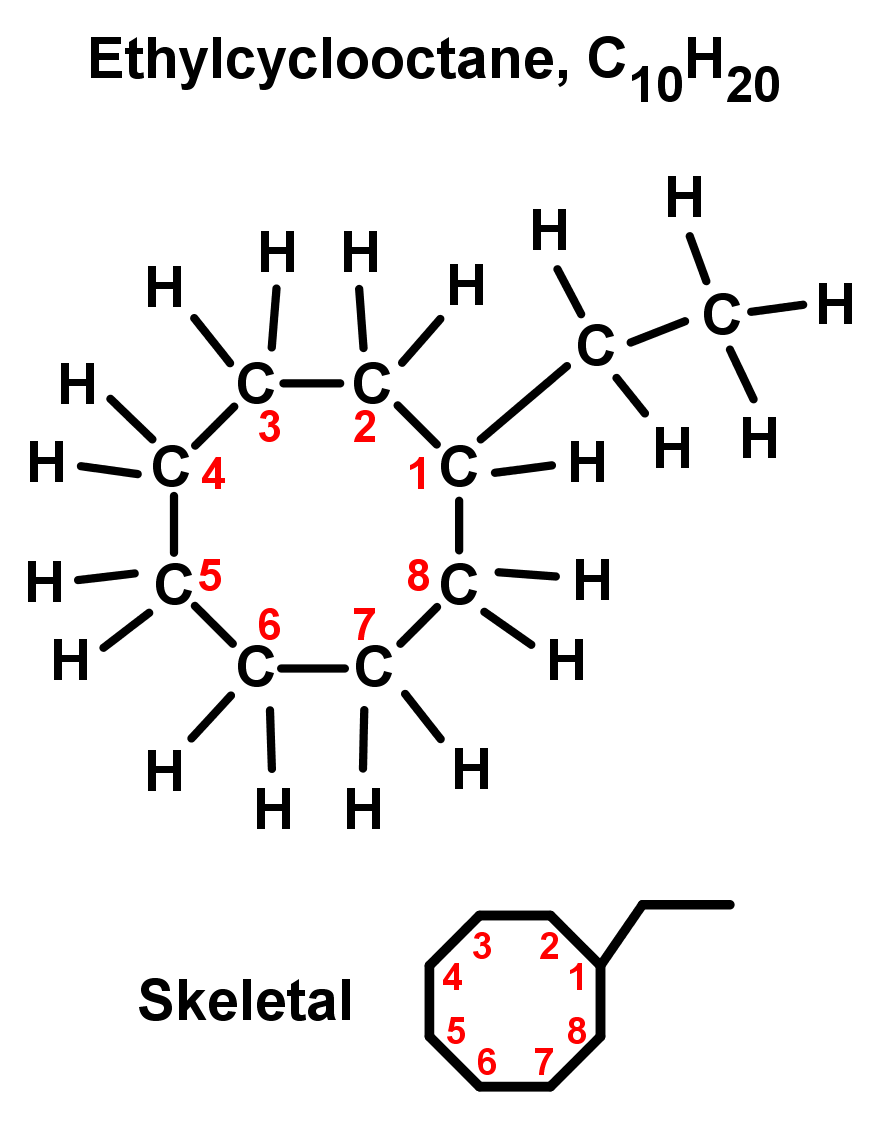
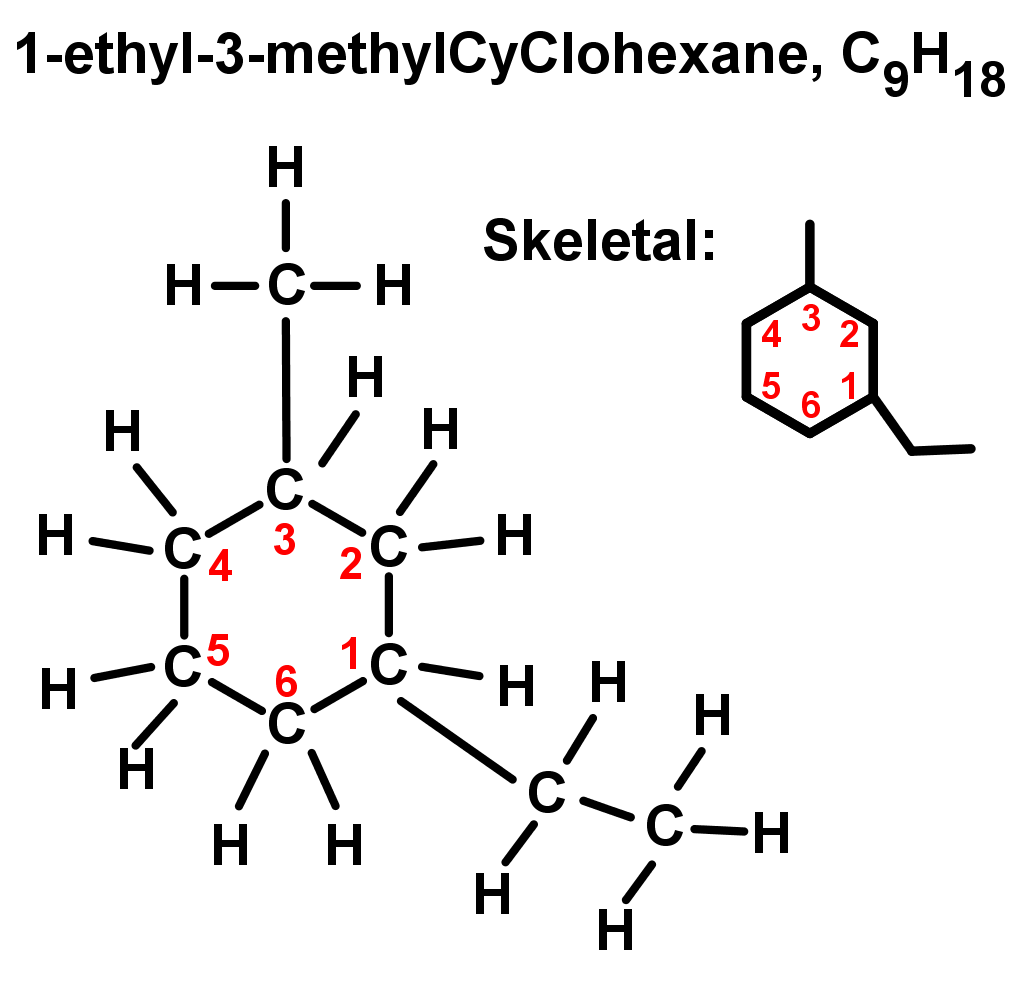