Ligands and complex ions
![]() All You Need in One PlaceEverything you need for better marks in primary, GCSE, and A-level classes. | ![]() Learn with ConfidenceWe’ve mastered the UK’s national curriculum so you can study with confidence. | ![]() Instant and Unlimited Help24/7 access to the best tips, walkthroughs, and practice questions. |
Make math click 🤔 and get better grades! 💯Join for Free
0/5
Intros
Lessons
0/4
Examples
Lessons
- Explain the difference between colour appearances in d-block compounds.
- Understand How ligands affect coordination number due to denticity and size.
Free to Join!
StudyPug is a learning help platform covering maths and science from primary all the way to second year university. Our video tutorials, unlimited practice problems, and step-by-step explanations provide you or your child with all the help you need to master concepts. On top of that, it's fun — with achievements, customizable avatars, and awards to keep you motivated.
Easily See Your Progress
We track the progress you've made on a topic so you know what you've done. From the course view you can easily see what topics have what and the progress you've made on them. Fill the rings to completely master that section or mouse over the icon to see more details.Make Use of Our Learning Aids
Earn Achievements as You Learn
Make the most of your time as you use StudyPug to help you achieve your goals. Earn fun little badges the more you watch, practice, and use our service.Create and Customize Your Avatar
Play with our fun little avatar builder to create and customize your own avatar on StudyPug. Choose your face, eye colour, hair colour and style, and background. Unlock more options the more you use StudyPug.
Topic Notes
In this lesson, we will learn:
- The definition of complex ion, ligand and coordination number.
- How to name simple ligands and how they bond to metals to create complex ions.
- The conditions that create coloured transition metal complexes.
- How ligand size and denticity affects the coordination number of a complex ion.
- Some important examples of complex ions and mutidentate ligands.
- In the last lesson Introduction to transition metals and the d-block, we learned that transition metals form coloured compounds and have variable oxidation states. The colour doesnt come from the metal ions alone; it occurs when metal ions form compounds with other molecules bonding to them, forming what we call a coordination complex or a complex ion.
- Complex ions are made of a central metal ion(s) surrounded by ligands bonding to the metal via dative bonding. In dative (coordinate) bonding both electrons are donated by one species, which here is the ligand.
Since dative bonds have both electrons coming from one atom in the covalent bond, molecules/atoms with lone pairs are very common ligands. The most common is probably water (H2O:) and ammonia (:NH3) is also very common. See the image below for an example of dative bonding. - A ligand is any molecule that datively bonds to a metal centre using a lone pair. There are many different ligands anything that can donate a lone pair could be a ligand:
- Neutral molecules with lone pairs, such as water, are very common ligands. When water acts as a ligand it is called aqua in the compounds name. Ammonia (NH3) is another common ligand, called ammine in complex ions.
For example, the compound below is called iron (II) hexaaqua, for the six molecules datively bonding to the one metal centre. - Anions can also act as ligands by donating a lone pair. An example of this is the cobalt (II) chloride complex. See the image below:
- Ligands bond to metal centres using EMPTY orbitals. They do not interact with the partially filled 3d orbitals that already contain electrons.
This is because the ligands are contributing two electrons to make the bond. They cannot fit into an orbital that already contains an electron. - Ligands cause splitting of the metals d-orbitals into two sections with different energy. The energy gap between these sections corresponds to the visible spectrum, which causes the colour of the compound to appear..
Before the ligands bond, the five d-orbitals are all equal energy (known as degenerate orbitals). But when the ligands interact with the empty higher energy orbitals, it causes a splitting in the partially filled d-subshell.
There are several different splitting patterns that can occur. We are just focusing on one type called octahedral splitting. - For example, the Fe2+ ion has the electron configuration [Ar] 4s0 3d6, with one d-orbital holding a pair of electrons and the other four with one electron each. When mixed with water to become [Fe(H2O)6]2+, the dative bonds from the water ligands cause the five d-orbitals to split. Now, three are lower energy than before, and two are higher.
In this state, an electron in a lower-energy d orbital can go from its current orbital to a higher energy d-orbital by absorbing visible light (photons with frequency in the visible region).
This is called a d-d transition and the electron is absorbing colour when it does this. The colour we observe is complementary to the colour absorbed by the electrons.
This is why transition metals produce colour in complexes! - Different ligands have different strength in their interactions, so they produce different d-orbital splitting and therefore a different ΔE (energy gap) between the lower and higher d-orbitals. This will correspond to a different wavelength of light to be absorbed and make a d-d transition. In short, changing the ligand will change the observed colour of the complex ion.
See below for a comparison between [Fe(H2O)6]2+ and [Fe(CN)6]4-. - If the d-orbitals are completely empty (like in Sc), there are no electrons to make d-d transitions, and the ligands interact with those empty orbitals instead.
- If the d-subshell is completely full (like in Zn), there is no space in an empty or partially filled orbital for a d-d electron transition to happen.
- The oxidation number and charge of the metal is also responsible for different coloured complex ions. The differing oxidation number will affect the strength of ligand interactions and lead to a different △E.
- The number of ligands around a metal ion can affect the colour produced too. The number of ligands around a metal centre is called the coordination number.
- How many ligands can fit around a metal centre depends on their size chloride ligands for example are larger than water ligands. As a result, a metal centre can normally only accommodate four chloride ligands instead of six water ligands. This then affects the overall geometry of the complex:
- Complex ions with a coordination number of 6 usually have an octahedral geometry. This is the geometry taken with hexaaqua and hexaammine complex ions like [Co(H2O)6]2+.
- Complex ions with a coordination number of 4 usually have a tetrahedral geometry. This is the geometry taken with four chloride ligands, for example [CoCl4]2-.
- Some complex ions, due to the metal ion, can form square planar complexes with a coordination number of four. This is the geometry of cis-platin, a platinum-based anticancer drug.
Importantly, the anticancer properties are only found in the cis-isomer, not the trans isomer where the two NH3 ligands are opposite each other. - Another factor that affects coordination number is denticity this is the number of dative bonds that the ligand makes to the metal centre. Most ligands are monodentate ligands which means only one dative bond is made, but there are many multidentate ligands. See the table below for some common multidentate ligands.
- As well as cis-platin, the haemoglobin molecule (the oxygen-carrying agent in our blood) is a coordination complex. It is an iron (II) based complex with a multidentate ligand that carries oxygen because O2 binds as a ligand to the Fe2+ ion. It is a relatively weak interaction. Thats good; it means the O2 ligand can leave and oxygen can get into our cells!
Unfortunately, ligands binding to haemoglobin is exactly why carbon monoxide (CO) is highly toxic to humans. CO is far stronger than O2 at binding to haemoglobin, so CO molecules will replace O2 ligands on the haemoglobin complex and prevent O2 from being transported in our body.
This type of reaction is called a ligand exchange which well look at next lesson!
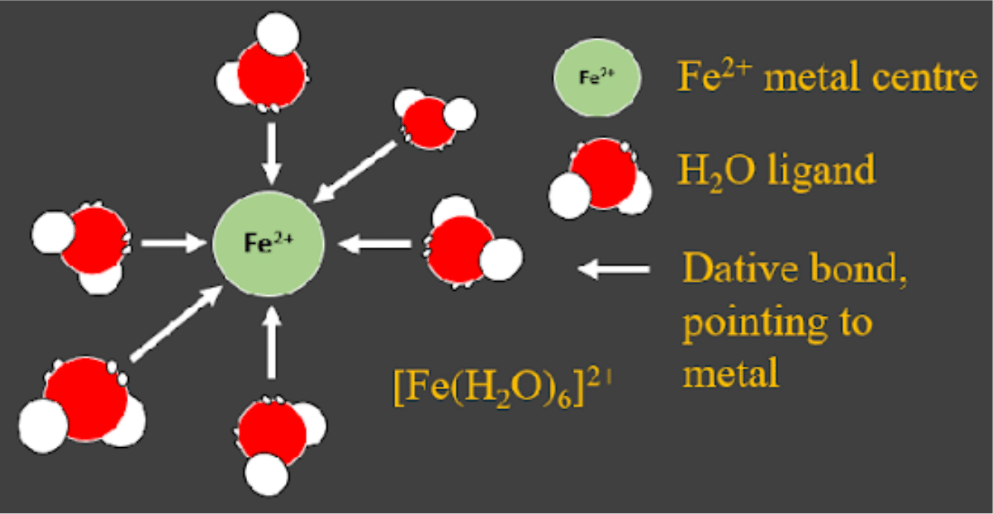
This is sometimes called dative covalent bonding, because the electrons are shared (the covalent part) despite both coming from one source.
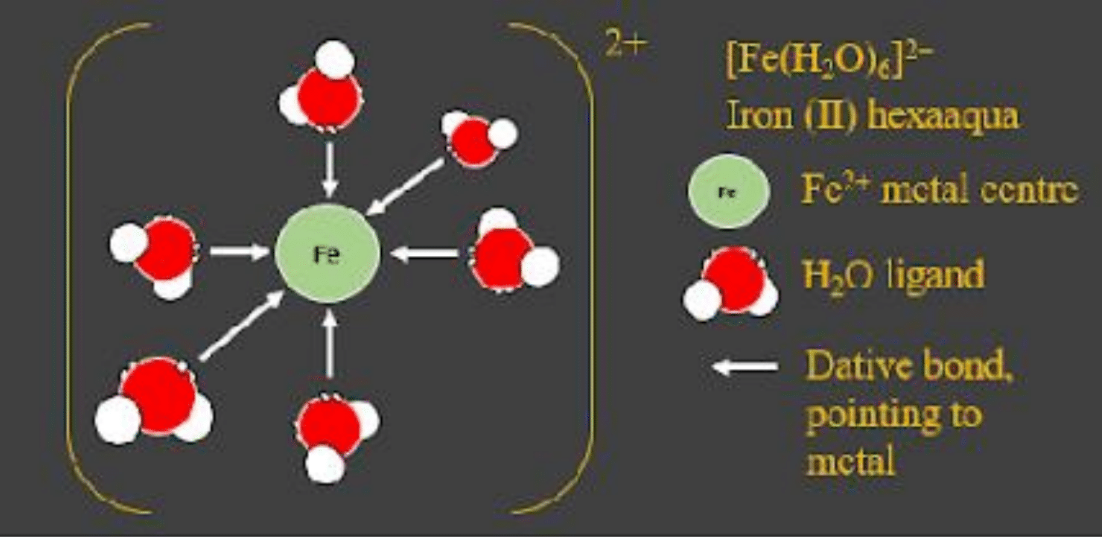
The charge on the metal and ligand will combine, just like in any other bonding. Since water is neutral, there isnt a change in charge from what the iron was before: Fe3+.
If its more than one atom, the ligand is written in regular brackets with the entire compound in square brackets, the charge outside. This is shown in the diagram as [Fe(H2O)6]3+.
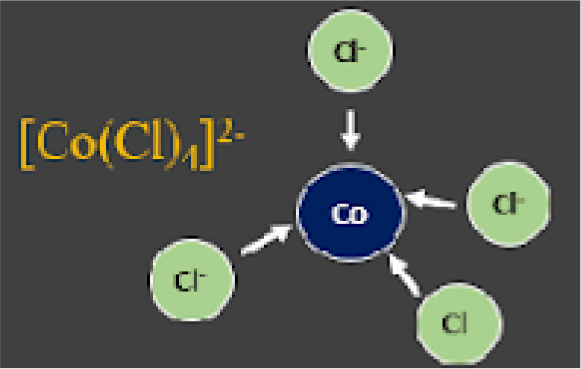
As chloride is a negatively charged species, their dative bonding to the Co2+ ion leads to an overall 4- charge for the complex, the result of Co2+ and 4Cl- bonding together. This is written [CoCl4]2-.
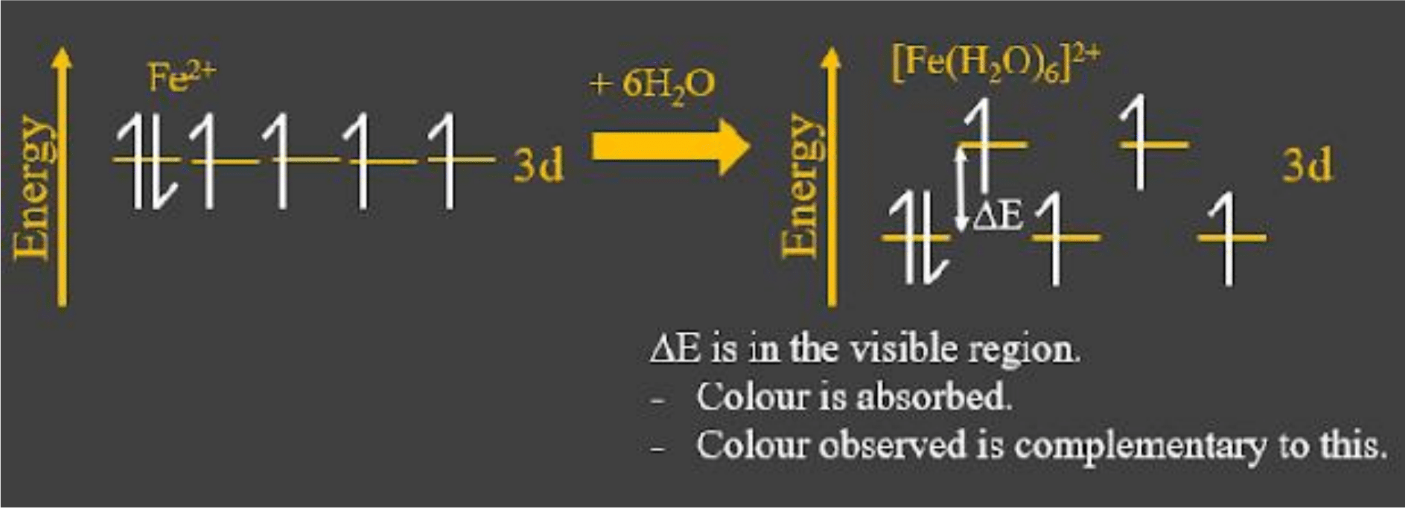
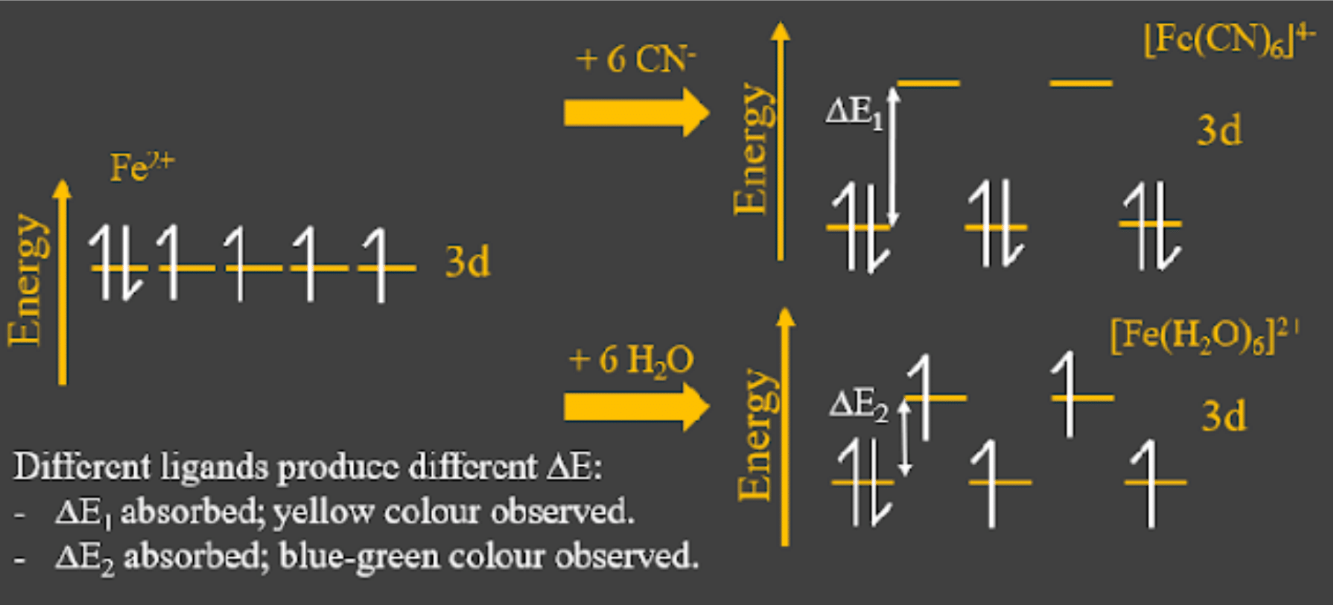
This is why metals that are not transition metals (partially filled d-orbitals) have colourless salts and solutions.
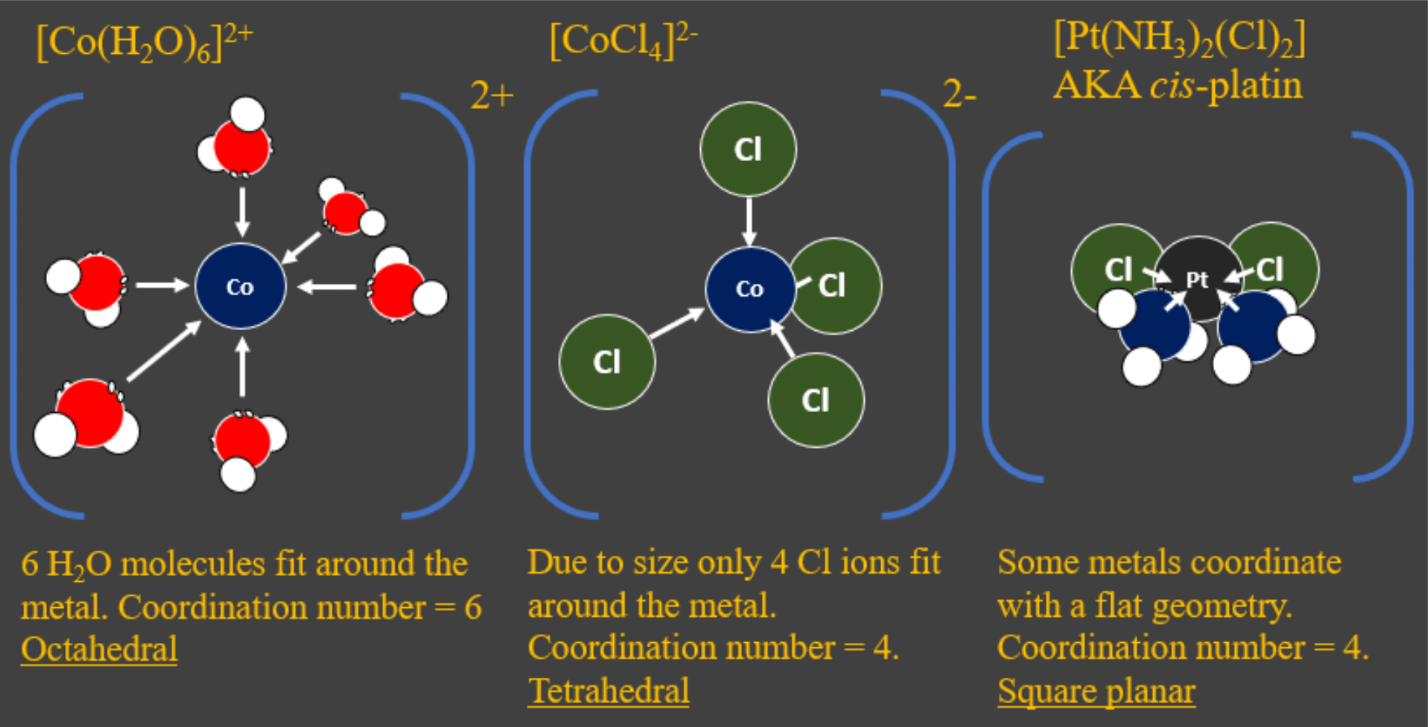
2
videos
remaining today
remaining today
5
practice questions
remaining today
remaining today