In this lesson, we will learn:
- Solenoids
- Electromagnets
- Application on electromagnets and Solenoids
Notes:
Solenoid
- A long coil of wire consisting of many loops (or turns) of wire is called Solenoid.
- The magnetic field within the solenoid is quite large since is the sum of the fields due to the current in each loop.
- A solenoid acts like a magnet; one end can be considered the north pole and the other the south pole, depending on the direction of the current in the solenoid.
- Using the right-hand rule, we can distinguish the north and south pole;
- A long coil of wire consisting of many loops (or turns) of wire is called Solenoid.
- The magnetic field within the solenoid is quite large since is the sum of the fields due to the current in each loop.
- A solenoid acts like a magnet; one end can be considered the north pole and the other the south pole, depending on the direction of the current in the solenoid.
- Using the right-hand rule, we can distinguish the north and south pole;
- Wrap the right hand around the solenoid with the fingers in the direction of the conventional current.
- The thumb points in the direction of the magnetic north pole. (thumb points “N” pole).
- The following equation is applied to calculate the magnitude of the magnetic field of a solenoid;
- Iron-core solenoid: A piece of iron is placed inside a solenoid; the magnetic field is increased greatly because the iron becomes a magnet.
- The resulting magnetic field is the sum of that due to the current and that due to the iron and can be hundreds or thousands of times that due to the current alone.
- Applications;
- Electromagnets are used in motors and generators to produce large magnetic fields.
- Electromagnets are used as switches and circuit breakers.
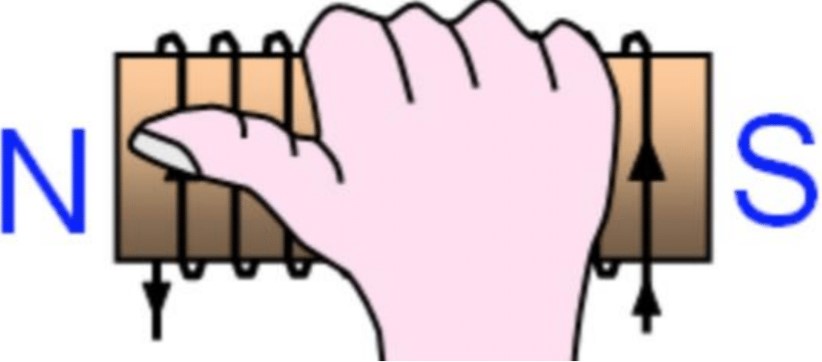
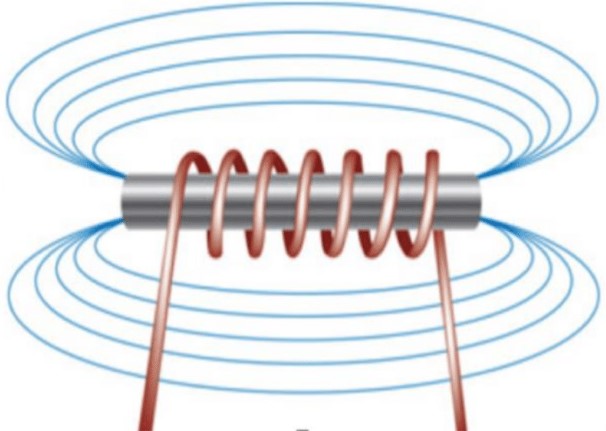