In this lesson, we will learn:
- Galilean-Newtonian relativity
- Inertial frame of references Vs. Non-inertial frame of references
- Relativity principles
- Comparison between variables among different frame of references
- The Michelson-Morley experiment
- Postulates of the Special Theory of Relativity
- Time Dilation and Twin Paradox.
Notes:
Galilean-Newtonian relativity
This theory deals with observing objects and events from different frame of references.
The special relativity deals with the “inertial frame of reference” in which the Newton’s first law of motion is valid. It is easier to measure and observe events using the inertial frame of reference.
Inertial frame of references Vs. Non-inertial frame of references
Although Earth is rotating, for most purposes we take the earth as inertial frame of reference
A frame of reference moving at constant speed with respect to an inertial frame is also considered as an inertial frame of reference.
Any rotating or accelerating frame is considered as a non-inertial frame of reference.
Relativity principles
The laws of physics are the same in all inertial frame of reference;
Examples: while travelling in a train, which moves with constant velocity, bodies move just as just they do when you are at rest on the Earth.
Relativity principles
The laws of physics are the same in all inertial frame of reference;
Examples: while travelling in a train, which moves with constant velocity, bodies move just as just they do when you are at rest on the Earth.
Dropping a coin inside the car and taking two different frame of references;
- Frame of reference: car
The coin falls straight downward and hit the floor directly below the point of release. - Frame of reference: Earth
From an observer point of view on the Erath, the coin covers a curved path.
Conclusion;
The actual path covered by the coin is different as viewed from different frames of references.
This does not contradict the laws of physics, the same law of gravity and motion applies in both reference frames. (same acceleration in both frame of references)
The only difference;
In the first frame of reference the initial velocity of the coin is zero but in the second frame of reference the coin has same initial velocity as the car.
In classical mechanics, space and time intervals are considered to be absolute;
In any frame of reference, the TIME INTERVAL, LENGTH, is the same. Their measurement does not change form one reference frame to another.
Mass of the object and all forces are assumed to be the same in all frame of references.
Comparison between variables among different frame of references
|
|
|
|
|
|
|
|
|
|
|
|
|
|
|
|
|
|
|
|
|
|
|
|
Example
A person walks inside a train toward the front with a speed of 4m/s. but if the train moves 20m/s with respect to the Earth, then the person is moving with 24m/s with respect to the Earth. The acceleration, however, is the same for both frame of references.
Frame of reference; train
The person accelerates from 0 to 4m/s in 2 seconds
Frame of reference; the Earth
The person accelerates from 20 to 24 in 2 seconds
According to Newton’s second law;
Acceleration is constant
Mass is constant
Therefore, force does not change as the frame of reference changes.
In general, we can say, all inertial frames are equivalent, laws of mechanics are the same in any initial frame of reference.
The “ether”
Waves can travel trough medium, such as; water, air, rope.
The medium though which light waves can travel is called the “ether”.
A transparent medium with zero density through which light can travel.
Maxwell’s predicted that light is an electromagnetic wave, using his equation the velocity of light calculated to be 3.0×108m/s, but is equation has no provision for relative velocity. (no specific frame of reference is defined by Maxwell) Scientists assume that the Maxwell’s equitation must be with respect to the ether.
The Michelson-Morley experiment
The Michelson-Morley experiment was done to check the existence of the ether.
The difference in the speed of light in different directions is measured.
They were expecting to find different values for the speed of light as the orientation of the apparatus was changing. The existence of the ether should affect the velocity of the light in different directions.
Null result: no difference was detected
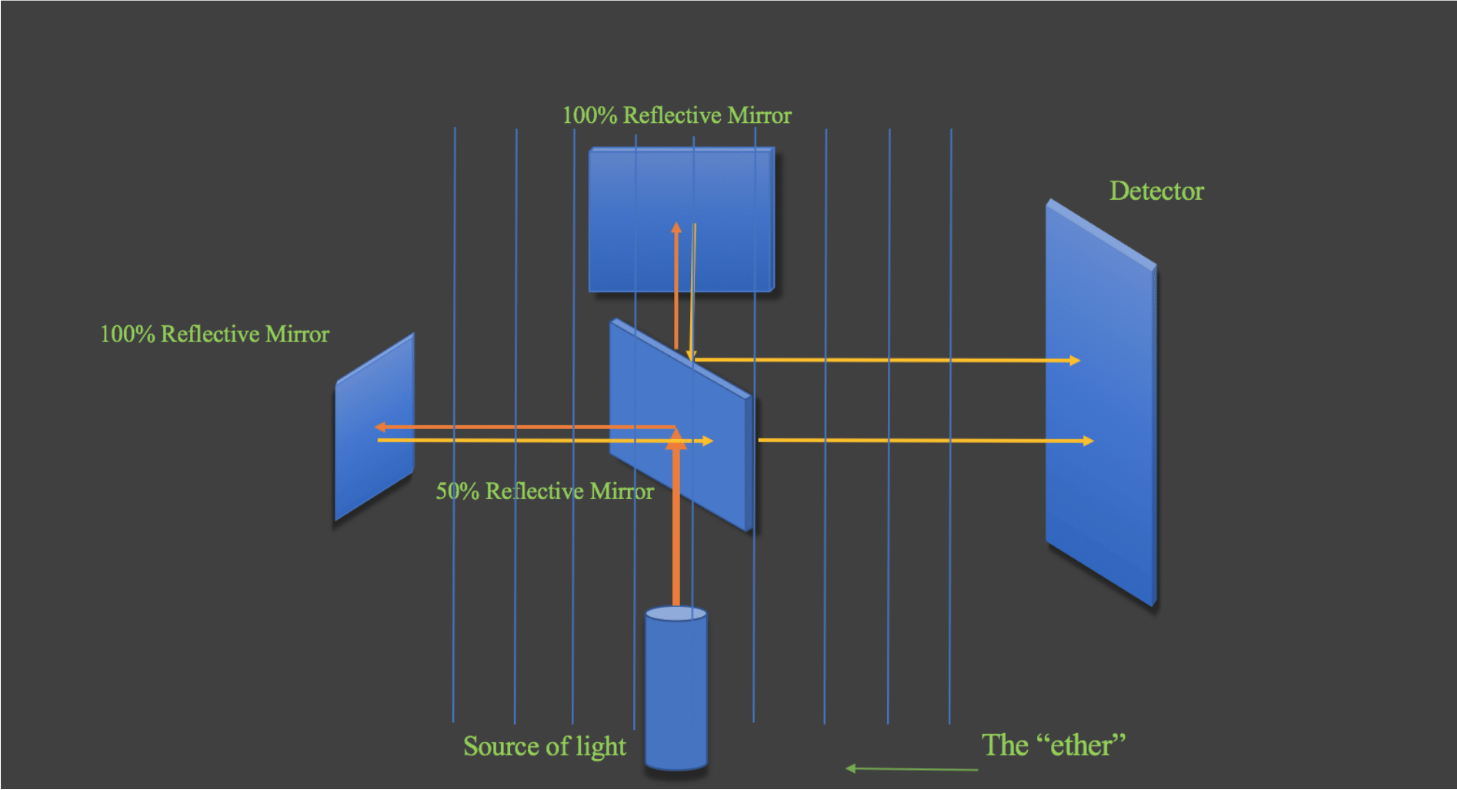
Postulates of the Special Theory of Relativity
The postulates proposed by Einstein is to come up with a solution to the “ether” existence.
The first postulate
The laws of physics (not only the laws of mechanics) have the same form in all inertial reference frames.
The second postulate
Light propagates through empty space with a definite speed c independent of the speed of the source or observer.
(The speed of light predicted by Maxwell’s equation, is the speed of light in vacuum in any frame of reference)
Note: The special theory of relativity, deals only with inertial frames.
Time Dilation and Twin Paradox
To calculate the time Dilation, lets calculate the time taken for light to travel across a spaceship and back from two different observers;
- Observer on the spaceship
- Observer on the Earth
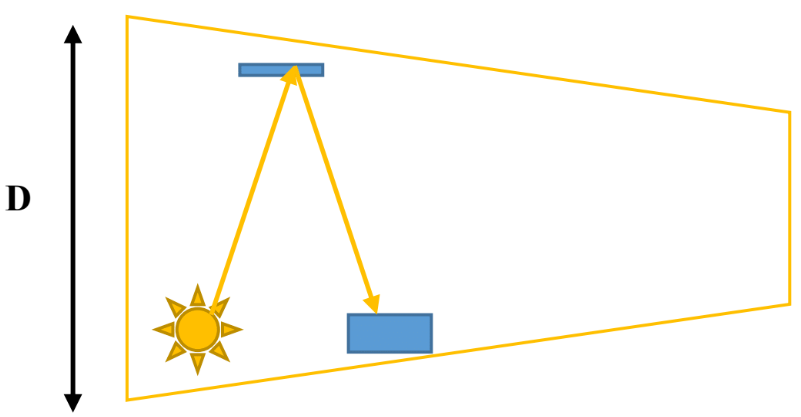
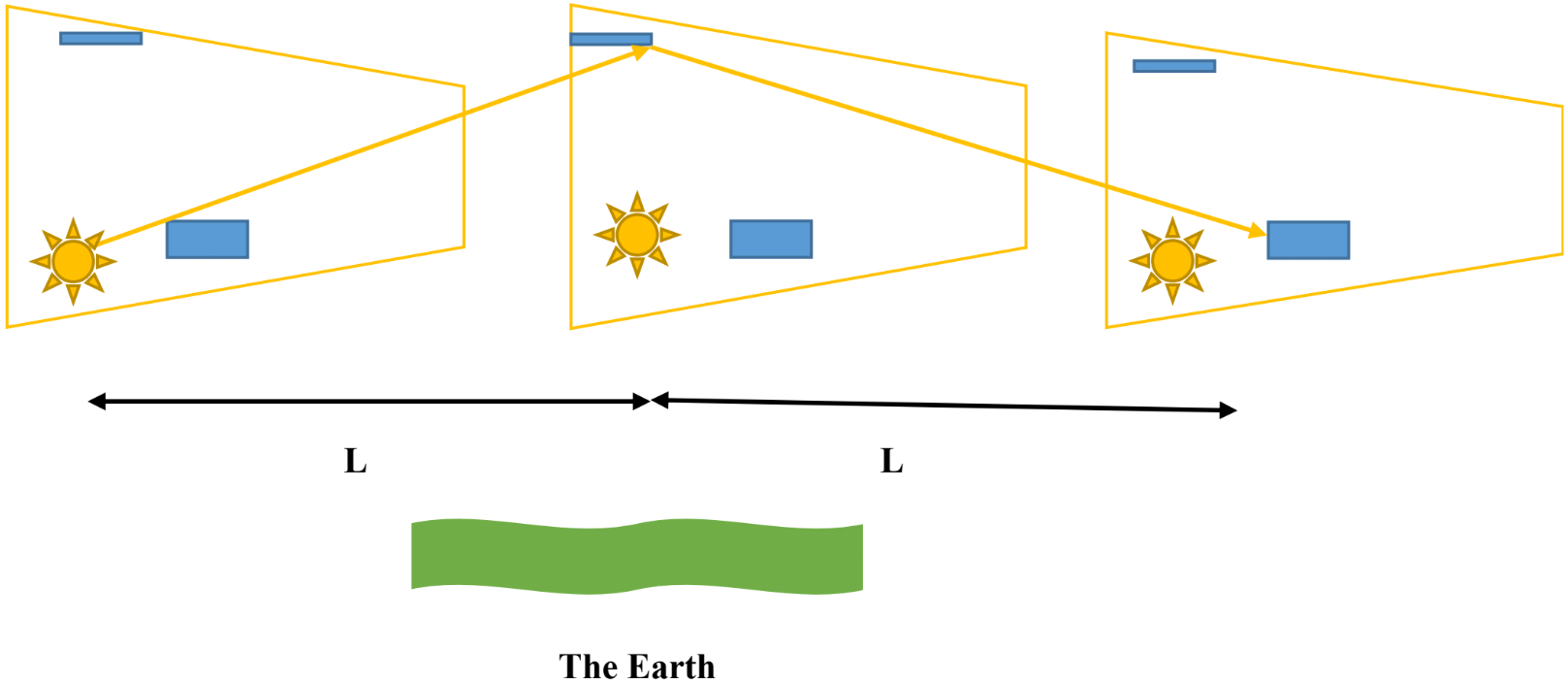
is the speed of the spaceship
the time interval, t, observed by the observer on the Earth can be calculated as follows;
The light ravels the total distance on its diagonal path (Pythagoras theorem), therefore,
distance travelled by light is 2
distance travelled by light can be calculated using;
. (distance travelled by light)
but, 2
2 where 2 2
We square both sides,
In the reference frame of the spaceship, the time interval measured by observer on spaceship is calculated as
Substituting 2D in equation we can find in terms of and .

: the time interval observed by the observer on Earth
: the time interval observed by the observer on Earth
: the speed of the spaceship
: the speed of light
Note:
Always < is always more than one >
Time Dilation equation can also be written as; where,
The fact that two events simultaneous to one observer may not be simultaneous to a second observer, suggest that time is not absolute.
Twin paradox
According to the twin paradox, if a pair of 20-year-old twins are separated, one takes off to the space with a spaceship travelling at very high speed, and the other one stays on the Earth. The astronaut tween will age lese. Whereas 20 years might pass for the Earth twin, the astronaut, perhaps only one year would pass for the traveler. When the traveler returns, the earthbound twin would be 40 years old whereas the traveling twin would be only 21.
As the speed of a moving object gets closer to the speed of the light, the slower the time travels.