Light and matter
Intros
Lessons
Examples
Lessons
- Give the reason why would you choose a window shade that is translucent or opaque.
- What color do you add to green light to obtain white light?
- What primary pigment colors must be mixed to get blue?
- What color will a yellow tennis ball appear when is illuminated by
- White light
- Green and red light
- Blue
- What would be the result of passing a beam of light through polarizing filter?
- Can sound waves be polarized?
Free to Join!
Easily See Your Progress
We track the progress you've made on a topic so you know what you've done. From the course view you can easily see what topics have what and the progress you've made on them. Fill the rings to completely master that section or mouse over the icon to see more details.Make Use of Our Learning Aids
Earn Achievements as You Learn
Make the most of your time as you use StudyPug to help you achieve your goals. Earn fun little badges the more you watch, practice, and use our service.Create and Customize Your Avatar
Play with our fun little avatar builder to create and customize your own avatar on StudyPug. Choose your face, eye colour, hair colour and style, and background. Unlock more options the more you use StudyPug.
Topic Notes
Introduction to Light and Matter
Welcome to our exploration of Light and Matter! This fascinating topic is at the heart of understanding how our universe works. As we dive in, you'll discover the incredible properties of light and its interactions with matter. Our journey begins with an engaging introduction video that sets the stage for our learning adventure. This video is a crucial starting point, offering a visual and dynamic way to grasp these complex concepts. It breaks down the basics, making them accessible and exciting. As your math tutor, I'm thrilled to guide you through this material. We'll uncover how light behaves both as a wave and a particle, and explore its unique relationship with different types of matter. From reflection and refraction to absorption and emission, we'll see how light's properties shape our world. This knowledge forms the foundation for understanding more advanced topics in physics and beyond. Let's embark on this illuminating journey together!
Transmission of Light Through Materials
When it comes to understanding how light interacts with different materials, we often encounter three main categories: transparent, translucent, and opaque. These terms describe how light passes through (or doesn't pass through) various substances, and they play a crucial role in our everyday experiences with light and vision.
Let's start with transparent materials. These are substances that allow light to pass through them almost completely, with very little absorption or scattering. The most common example of a transparent material is clear glass. When you look through a clean window, you can see objects on the other side with remarkable clarity. This is because the light rays from those objects pass through the glass virtually unimpeded. Other examples of transparent materials include certain plastics, pure water, and some crystals.
The key characteristic of transparent materials is that they maintain the integrity of images passing through them. When you look at a landscape through a transparent window, the image you see is essentially unchanged. This property makes transparent materials ideal for applications where clear visibility is crucial, such as in eyeglasses, camera lenses, and car windshields.
Moving on to translucent materials, we enter a fascinating middle ground between transparency and opacity. Translucent substances allow light to pass through, but they scatter it in the process. This scattering effect means that while light can penetrate the material, images become blurred or distorted. A perfect example of a translucent material is frosted glass. When you look through frosted glass, you can perceive light and vague shapes, but you can't make out clear details.
Other examples of translucent materials include certain types of plastic, wax paper, and some fabrics like thin curtains. The degree of translucency can vary, with some materials allowing more light and image clarity than others. This property makes translucent materials useful in situations where we want to allow light while maintaining privacy, such as in bathroom windows or lampshades.
The effect of translucent materials on images is quite interesting. While the overall brightness might be preserved, the details become fuzzy. This can create a soft, diffused light effect that's often desirable in photography or interior design. However, it's important to note that the more translucent a material is, the more it will distort the images passing through it.
Finally, we come to opaque materials. These substances block light completely, preventing it from passing through. Wood is an excellent example of an opaque material. When you hold up a wooden board, no light passes through it, and you can't see anything on the other side. Other common opaque materials include metals, ceramics, and thick fabrics.
Opaque materials absorb or reflect all the light that hits them, depending on their surface properties. A black opaque object, for instance, absorbs most of the light, while a white opaque object reflects most of it. This property makes opaque materials crucial in applications where light blocking is necessary, such as in curtains for darkening a room or in light-sensitive photographic processes.
The interaction between light and these different types of materials has significant implications for image formation and perception. Transparent materials allow for clear image transmission, making them ideal for situations where accurate visual information is crucial. Translucent materials create a balance between light transmission and privacy, often resulting in softened or blurred images. Opaque materials, on the other hand, completely block image transmission, which can be useful for creating contrast or controlling light in specific environments.
Understanding these properties is not just academically interesting; it has practical applications in various fields. Architects and designers use the interplay of transparent, translucent, and opaque materials to control light and create desired atmospheres in buildings. Photographers manipulate these properties to achieve different lighting effects in their images. Even in everyday life, we make choices based on these properties, such as selecting curtains for our homes or choosing the right type of glass for our car windows.
In conclusion, the way light interacts with transparent, translucent, and opaque materials fundamentally shapes our visual world. From the clarity of a glass window to the soft glow of a frosted lamp shade to the light-blocking properties of thick curtains, these materials play a vital role in how we experience and control light in our environment. By understanding these concepts, we can make more informed decisions about the materials we use in various applications, whether for practical purposes or aesthetic effects.
Spectrum of Light and Prism
Have you ever marveled at the beauty of a rainbow arching across the sky after a rainstorm? That colorful display is nature's way of showing us the spectacular spectrum of light. The spectrum of light is a fascinating phenomenon that reveals the hidden colors within what we typically perceive as white light. It's like a secret code of colors waiting to be unlocked, and today, we're going to explore how we can observe and create this magical display.
First, let's define what a spectrum is. In simple terms, a spectrum is the range of colors that make up visible light. When white light is separated into its component colors, we see the full spectrum. This separation occurs naturally in a rainbow and can also be recreated in a laboratory setting using a prism.
The visible light spectrum consists of seven primary colors: red, orange, yellow, green, blue, indigo, and violet. You might remember these colors by the mnemonic ROY G. BIV. Each color in the spectrum has a different wavelength, with red having the longest wavelength and violet the shortest.
Now, let's talk about how we can observe this spectrum in nature. The most common and breathtaking example is the rainbow. When sunlight enters a raindrop, it slows down and bends (refracts). This bending causes the white light to separate into its component colors. As the light exits the raindrop, it reflects off the back of the drop and disperses into the sky, creating the arc of colors we see as a rainbow.
But what if we want to see the spectrum of light on a sunny day without waiting for rain? That's where the prism comes in. A prism is a transparent optical element with flat, polished surfaces that refract light. When white light passes through a prism, it behaves similarly to light passing through a raindrop. The prism slows down the light and bends it, separating the white light into its component colors.
In a laboratory setting, scientists and students can use a prism to study the properties of light. By shining a beam of white light through a prism, they can project a spectrum onto a screen or wall, allowing for detailed observation and measurement of the different wavelengths of light.
The study of light spectra has numerous practical applications. For instance, astronomers use spectroscopy to analyze the light from distant stars and galaxies, determining their composition and movement. In everyday life, we encounter spectra in things like CDs and DVDs, which use the principles of light diffraction to store and read data.
Understanding the spectrum of light helps us appreciate the complexity and beauty of the world around us. From the vibrant colors of a sunset to the twinkling of stars in the night sky, the spectrum of light plays a crucial role in how we perceive and interact with our environment.
So, the next time you see a rainbow or play with a prism, remember that you're witnessing one of nature's most beautiful phenomena the spectrum of light. It's a reminder of the hidden wonders that surround us, waiting to be discovered and understood. Whether observed in the sky after a rainstorm or created in a classroom with a simple prism, the spectrum of light continues to inspire curiosity and awe in scientists and nature lovers alike.
Additive Color Mixing
Additive color mixing is a fascinating concept that forms the basis of how we perceive colors in digital displays and light-based technologies. At its core, this process involves combining different colored lights to create new colors, with the ultimate goal of producing white light when all primary colors are mixed in equal proportions.
The foundation of additive color mixing lies in the three primary colors: red, green, and blue (RGB). These colors are considered primary because they cannot be created by mixing other colors of light. Instead, they serve as the building blocks for all other colors in the additive color system.
When we combine these primary colors in various intensities, we can create a wide spectrum of colors:
- Red + Green = Yellow
- Red + Blue = Magenta
- Green + Blue = Cyan
- Red + Green + Blue = White
The colors created by mixing two primary colors are known as secondary colors. In the additive color system, these secondary colors are yellow, magenta, and cyan. It's important to note that these secondary colors are different from those in the subtractive color system used in printing (which uses cyan, magenta, and yellow as primaries).
One of the most intriguing aspects of additive color mixing is the creation of white light. When all three primary colors - red, green, and blue - are combined in equal intensities, the result is white. This principle is utilized in various technologies, from stage lighting to digital screens, where tiny red, green, and blue light-emitting elements work together to produce a full range of colors, including white.
Complementary colors play a significant role in additive color mixing. In this system, complementary colors are pairs of colors that, when combined, produce white light. The complementary color pairs in the additive system are:
- Red and Cyan
- Green and Magenta
- Blue and Yellow
Understanding complementary colors is crucial in various fields, including graphic design, photography, and digital imaging. They can be used to create striking contrasts, enhance visual appeal, and achieve color balance in compositions.
The concept of additive color mixing has numerous practical applications. For instance, in digital displays such as computer monitors, televisions, and smartphone screens, each pixel consists of red, green, and blue subpixels. By varying the intensity of each subpixel, these devices can produce millions of different colors, creating the vibrant images we see on our screens.
In stage lighting, additive color mixing allows lighting designers to create a vast array of atmospheric effects and color schemes by blending different colored lights. This technique is essential in setting the mood and enhancing the visual experience in theaters, concerts, and other live performances.
To better visualize additive color mixing, imagine three spotlights - one red, one green, and one blue - shining on a white surface. Where the red and green lights overlap, you'll see yellow. Where red and blue intersect, you'll observe magenta. The overlap of green and blue produces cyan. And at the center, where all three colors converge, you'll see white light.
It's worth noting that the additive color system is different from the subtractive color system used in printing and painting. While additive mixing deals with light, subtractive mixing involves pigments or dyes that absorb certain wavelengths of light and reflect others. This is why the primary colors in printing are cyan, magenta, and yellow (with black added for depth), which is often referred to as the CMYK color model.
In conclusion, additive color mixing is a fundamental concept in understanding how we perceive and create colors using light. From the basic principles of combining primary colors to create secondary colors and white light, to the practical applications in technology and art, this system plays a crucial role in our colorful world. By grasping these concepts, we can better appreciate the complexity and beauty of color in both natural and artificial environments.
Pigments and Dyes: Color by Subtraction
Hey there! Let's dive into the colorful world of pigments and dyes. You might think they're the same thing, but they actually work in different ways to create the vibrant colors we see around us. Let's break it down in a way that's easy to understand.
First, let's talk about pigments. These are tiny solid particles that reflect certain wavelengths of light while absorbing others. They create color through a process called subtraction. Imagine you're looking at a tomato. The reason it appears red is that the pigments in its skin absorb all the other colors of light and reflect only the red wavelengths back to your eyes. Cool, right?
Dyes, on the other hand, are different. They're actually soluble substances that can be dissolved in liquids. When you dye a piece of fabric, the dye molecules bond with the fibers of the material. Like pigments, dyes also create color through subtraction, but they do it at a molecular level rather than with solid particles.
Now, let's talk about primary pigments. In the world of pigments, we have three primary colors: cyan, magenta, and yellow. These are different from the primary colors you might be familiar with in light (red, green, and blue). When it comes to pigments, we're dealing with subtractive color mixing.
Here's how it works: Cyan pigments absorb red light and reflect blue and green. Magenta pigments absorb green light and reflect red and blue. Yellow pigments absorb blue light and reflect red and green. When you combine these primary pigments, they create secondary pigments by absorbing more wavelengths of light.
For example, if you mix cyan and yellow pigments, you get green. This is because cyan absorbs red light, yellow absorbs blue light, and together they reflect only green light. It's like a team effort to create new colors!
Let's go back to our tomato example to see how this works in nature. The skin of a ripe tomato contains pigments called carotenoids, primarily lycopene. These pigments absorb blue and green light while reflecting red light, giving the tomato its characteristic red color. If you've ever seen a green tomato, that's because it contains more chlorophyll, which absorbs red and blue light while reflecting green.
Understanding how pigments and dyes work is crucial in many fields, from art and design to biology and chemistry. Artists use this knowledge to mix paints and create stunning visual effects. In nature, pigments play vital roles in photosynthesis, camouflage, and even attracting pollinators.
It's fascinating to think about how the colors we see are actually the result of light reflection being absorbed and reflected. The next time you look at a colorful object, whether it's a flower, a painting, or even your favorite t-shirt, you'll know that there's a complex process of light reflection and reflection happening right before your eyes.
Remember, in the world of pigments and dyes, color is all about subtraction. They selectively remove certain wavelengths of light, leaving only the colors we perceive. It's like a magic trick performed by nature and science, creating the vibrant world we see every day.
So, the next time you're admiring a beautiful sunset or choosing colors for your art project, take a moment to appreciate the incredible science behind what you're seeing. The interplay of light, pigments, and dyes is truly a wonder of our colorful world!
Thin Film Interference
Prepare to be dazzled by one of nature's most captivating light shows: thin film interference! This mesmerizing phenomenon is responsible for the shimmering, iridescent colors we see in soap bubbles, oil slicks, and even butterfly wings. It's a spectacular display that combines physics and art in the most beautiful way imaginable!
At its core, thin film interference occurs when light waves interact with extremely thin layers of transparent materials. The magic happens when light reflects off both the top and bottom surfaces of these films. As these reflected waves meet, they create a dazzling interplay of colors that can leave us spellbound.
Let's dive into the enchanting world of soap bubbles as a prime example. When sunlight hits a soap bubble, some light reflects off the outer surface, while some penetrates the thin film and reflects off the inner surface. As these light waves reunite, they either amplify or cancel each other out, depending on their phase. This is where the concepts of constructive and destructive interference come into play.
Constructive interference occurs when the reflected light waves align perfectly, reinforcing each other and creating vibrant colors. On the other hand, destructive interference happens when the waves are out of phase, canceling each other out and resulting in darker areas. The thickness of the film and the angle at which we view it determine which colors we see, creating a constantly shifting rainbow effect that's simply mesmerizing!
The same principle applies to oil films on water, where we often see swirling patterns of vivid colors. As the oil spreads and its thickness varies, different wavelengths of light undergo constructive interference, producing a breathtaking spectrum of hues that dance across the surface.
What's truly exciting about thin film interference is that it's not just a visual treat it has practical applications too! Scientists and engineers harness this phenomenon to create anti-reflective coatings for lenses, produce color-changing materials, and even develop advanced optical sensors. The possibilities are as colorful and diverse as the effect itself!
So, the next time you see a soap bubble floating by or notice the rainbow sheen on a puddle, take a moment to appreciate the incredible physics at play. Thin film interference is a reminder that the world around us is full of hidden wonders, just waiting to be discovered and admired. It's a celebration of light, color, and the intricate beauty of the natural world a true feast for both the eyes and the curious mind!
Polarization of Light
Light polarization is a fascinating phenomenon that plays a crucial role in our everyday lives, often without us even realizing it. At its core, polarization refers to the orientation of light waves as they travel through space. To understand this concept better, imagine light as a wave rippling along a rope. If you shake the rope up and down, you create vertical waves, while shaking it side to side produces horizontal waves. Light behaves similarly, oscillating in various directions perpendicular to its path of travel.
In nature, light from sources like the sun or a light bulb is typically unpolarized, meaning the waves vibrate in all directions. However, when light interacts with certain materials or surfaces, it can become polarized, with waves aligned in a specific direction. This is where the magic of polarization comes into play, especially in practical applications like polarized sunglasses.
Polarized sunglasses are perhaps the most well-known application of light polarization. These special lenses contain a polarizing filter, which acts like a microscopic venetian blind. This filter allows only light waves oriented in a particular direction to pass through while blocking waves in other orientations. As a result, polarized sunglasses can significantly reduce glare from reflective surfaces such as water, snow, or glass, providing clearer vision and enhanced comfort for the wearer.
The process of how polarizing materials work is quite intriguing. A polarizer is typically made of long molecules aligned in parallel rows. As light waves encounter this material, only the waves that vibrate in the same direction as the aligned molecules can pass through. Waves oriented perpendicular to the molecules are absorbed or reflected. This selective transmission of light waves is what gives polarizing materials their unique properties.
To visualize this, imagine two people holding a jump rope between them. If they shake the rope up and down, representing vertically polarized light, it can easily pass through a vertical slit in a fence (our polarizer). However, if they shake the rope side to side (horizontally polarized light), it won't fit through the same vertical slit. This simple analogy illustrates how a polarizer selectively filters light based on its orientation.
Beyond sunglasses, polarization finds applications in various fields. In photography, polarizing filters can enhance contrast, reduce reflections, and deepen the color of skies in outdoor shots. LCD screens in our devices use polarizers to control which pixels are illuminated, creating the images we see. In scientific research, polarization techniques are used in microscopy to study materials and biological samples at a molecular level.
The automotive industry also leverages polarization technology. Some car windshields incorporate a polarizing layer to reduce glare from oncoming headlights, improving safety during night driving. Additionally, 3D movie theaters use polarization to create the illusion of depth. By projecting two slightly different images with orthogonal polarizations and providing viewers with correspondingly polarized glasses, each eye receives a unique image, resulting in a three-dimensional visual experience.
Understanding light polarization opens up a world of possibilities for controlling and manipulating light. From enhancing our visual comfort to enabling cutting-edge technologies, the principles of polarization continue to shape our interaction with the world around us. As we delve deeper into the realm of optics and photonics, the applications of polarization are likely to expand, offering new solutions to challenges in fields ranging from telecommunications to quantum computing.
In conclusion, light polarization is a fundamental property of electromagnetic waves that has far-reaching implications in both natural phenomena and human-made technologies. By harnessing the power of polarized light, we've developed tools and techniques that enhance our daily lives, push the boundaries of scientific research, and pave the way for future innovations. The next time you put on a pair of polarized sunglasses or marvel at a glare-free display, remember the intricate dance of light waves that makes it all possible.
Conclusion
In this exploration of light and matter, we've uncovered fascinating insights into the fundamental nature of our universe. The introduction video served as a crucial foundation, illuminating the complex interplay between light and various forms of matter. We've learned how light behaves as both a wave and a particle, and how it interacts with different materials. The concepts of reflection, refraction, and absorption have been key to understanding these interactions. Moreover, we've seen how light's properties are harnessed in technologies ranging from fiber optics to solar panels. To deepen your understanding, consider exploring additional resources on quantum physics or conducting simple at-home experiments with prisms or diffraction gratings. The study of light and matter opens doors to countless scientific wonders, and we encourage you to continue your journey of discovery in this captivating field.
Light and Matter: Transmission of Light Through Matter
Understanding how light interacts with different materials is crucial in various fields, from physics to engineering. This guide will walk you through the transmission of light through matter, based on the properties of different materials.
Step 1: Introduction to Light Transmission
Light transmission refers to the passage of light waves through a material. When light encounters a material, it can be transmitted, absorbed, or reflected. The behavior of light depends on the properties of the material it interacts with. In this section, we will explore how light behaves when it passes through different types of materials.
Step 2: Types of Materials
Materials can be categorized based on how they interact with light. There are three main types of materials: transparent, translucent, and opaque. Each type has distinct characteristics that affect light transmission.
Step 3: Transparent Materials
Transparent materials allow light to pass through them without distorting the image. Examples of transparent materials include clear glass and air. When light passes through a transparent material, you can see objects clearly on the other side. For instance, if you place a letter behind a pane of glass, you will be able to see the letter clearly without any distortion.
Step 4: Translucent Materials
Translucent materials allow light to pass through, but they scatter the light in different directions, causing objects to appear blurry or distorted. Examples of translucent materials include frosted glass and frosted light bulbs. When light passes through a translucent material, you can recognize that there is an object on the other side, but you cannot see its details clearly. For example, if you place a letter behind frosted glass, you will see a blurry image of the letter.
Step 5: Opaque Materials
Opaque materials do not allow light to pass through them. Instead, they absorb or reflect all the light that hits them. Examples of opaque materials include wood and brick. When light encounters an opaque material, it is either absorbed by the material or reflected back. For instance, if you place a letter behind a block of wood, you will not be able to see the letter at all.
Step 6: Summary of Light Transmission
To summarize, the transmission of light through matter depends on the type of material:
- Transparent materials: Light passes through clearly, and the image is not distorted.
- Translucent materials: Light passes through but the image is blurry or distorted.
- Opaque materials: Light does not pass through; it is either absorbed or reflected.
Step 7: Practical Applications
Understanding the transmission of light through different materials has practical applications in various fields. For example, in architecture, choosing the right type of glass for windows can affect the amount of natural light entering a building. In photography, using translucent materials can create soft lighting effects. In manufacturing, selecting the appropriate materials can impact the efficiency of optical devices.
Step 8: Conclusion
In conclusion, the transmission of light through matter is a fundamental concept that helps us understand how light interacts with different materials. By categorizing materials as transparent, translucent, or opaque, we can predict how light will behave when it encounters these materials. This knowledge is essential for various applications in science, technology, and everyday life.
FAQs
-
What is the difference between transparent, translucent, and opaque materials?
Transparent materials allow light to pass through clearly, like glass. Translucent materials allow some light to pass through but scatter it, like frosted glass. Opaque materials block light completely, like wood or metal.
-
How does a prism create a spectrum of colors?
A prism separates white light into its component colors by refracting different wavelengths at slightly different angles. This process is called dispersion and results in the familiar rainbow spectrum.
-
What are the primary colors in additive color mixing?
The primary colors in additive color mixing are red, green, and blue (RGB). When these colors are combined in various intensities, they can create a wide range of colors, including white when all are mixed equally.
-
How do pigments and dyes create color?
Pigments and dyes create color through subtraction. They absorb certain wavelengths of light and reflect others. The reflected wavelengths determine the color we perceive. For example, a red pigment absorbs all colors except red, which it reflects.
-
What causes the colorful effect in soap bubbles?
The colorful effect in soap bubbles is caused by thin film interference. Light waves reflect off both the outer and inner surfaces of the bubble's thin film. These reflected waves interfere with each other, either amplifying or canceling out certain wavelengths, resulting in the vibrant, shifting colors we see.
Prerequisite Topics
Understanding the fundamental concepts that lay the groundwork for more advanced topics is crucial in the study of physics. When delving into the fascinating realm of light and matter, it's essential to have a solid grasp of certain prerequisite topics. One such critical concept is the reflection of light, which serves as a cornerstone for comprehending the intricate interactions between light and matter.
The reflection of light is a phenomenon that we encounter daily, from seeing our reflection in mirrors to observing the glimmer of sunlight on water surfaces. This basic principle plays a pivotal role in understanding how light behaves when it encounters different materials. By mastering the concepts of reflection of light, students can better appreciate the complexities of light-matter interactions.
When studying light and matter, the principles of reflection become indispensable. For instance, understanding how light reflects off various surfaces helps explain phenomena such as the formation of images in mirrors and the behavior of light in optical fibers. The laws of reflection, including the equality of angles of incidence and reflection, form the basis for more advanced topics in optics and photonics.
Moreover, the reflection of light is closely tied to the concept of wave-particle duality, a fundamental principle in quantum mechanics. As students progress in their study of light and matter, they'll discover how reflection contributes to our understanding of light's dual nature as both a wave and a particle.
In the context of matter, reflection principles help explain how different materials interact with light. For example, the reflective properties of metals, which are crucial in many technological applications, can be better understood through a solid foundation in light reflection. This knowledge extends to the development of advanced materials with specific optical properties, such as anti-reflective coatings or highly reflective surfaces used in solar panels and telescopes.
Furthermore, the study of reflection of light introduces students to important mathematical concepts and problem-solving techniques that are applicable across various areas of physics. These skills, including the use of trigonometry and vector analysis, are invaluable when tackling more complex problems in the field of light and matter interactions.
By thoroughly grasping the principles of light reflection, students build a strong foundation for exploring more advanced topics in optics, such as refraction, diffraction, and polarization. This knowledge is not only crucial for academic success but also for understanding real-world applications in fields like telecommunications, medical imaging, and cutting-edge display technologies.
In conclusion, the reflection of light serves as a vital prerequisite topic for the study of light and matter. Its importance cannot be overstated, as it provides the necessary groundwork for comprehending more complex phenomena and applications in modern physics and technology. Students who invest time in mastering this fundamental concept will find themselves well-equipped to tackle the challenges and opportunities that lie ahead in their exploration of light and matter.
In this lesson, we will learn:
- Transmission of light through different materials
- Formation of spectrum of light
- Additive color process
- Colors by subtraction
- Formation of color by light, pigments, and dyes
- Polarization of light
Notes:
- Transparent materials: Transmit light waves without distorting images (glass, air)
- Translucent materials: Transmit light waves but objects can not be see clearly (frosted light bulbs)
- Opaque: Transmit NO light but absorbs and reflects all light incident upon them (wood, brick)
- Spectrum: When a narrow beam of light passes though a prism, rainbow of different colors can be seen
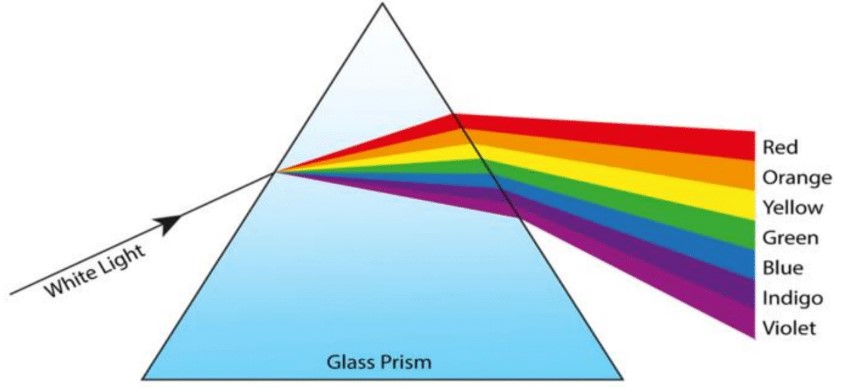
- Additive color process (RGB): The additive mixture of blue, green, and red light produces white light.
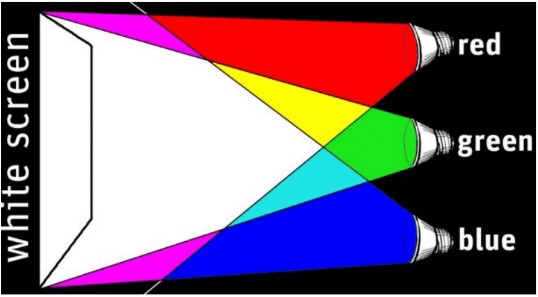
- Primary colors: red, green, blue
- Secondary colors: The primary colors are mixed by pairs to produce secondary colors;
- Yellow (red + green), Cyan (blue + green), Magenta (blue + red)
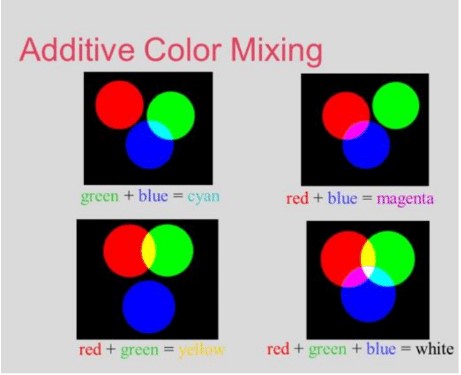
- Complementary colors: Secondary colors of light, when combined with the primary color, produces white light.
- Cyan is complementary to red
- Magenta is complementary to green
- Yellow is complementary to blue
Dye Vs. Pigment
- Dye: A molecule that absorbs certain wavelengths of light, and transmit or reflects others
- Example: Tomato is red because it reflects red and absorbs green and blue lights.
- Pigment: A colored material that absorbs a certain colors and transmits or reflects others. Pigment particles are larger than a molecule and can be seen with a microscope.
Primary Pigment Vs. Secondary Pigment
- Primary Pigment: A pigment that absorbs only ONE primary color from white light and reflects the other two. (yellow pigment absorbs blue and reflects green and red)
- Secondary Pigment: A pigment that absorbs TWO primary colors and reflects one. (red absorbs green and blue, green absorbs red and blue, blue absorbs red and green)
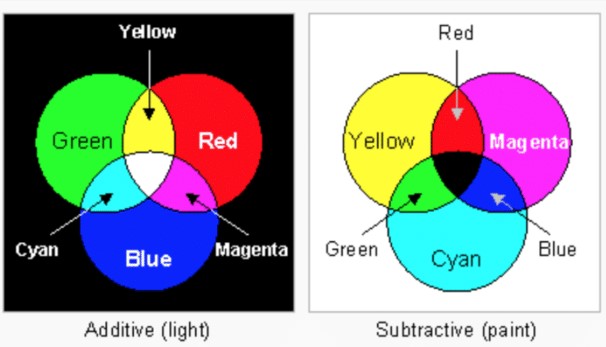
- Thin-film interference: Spectrum of colors produced by a soap bubble or by the oily film on water. Colors formation is the result of constructive and destructive interference of light waves.
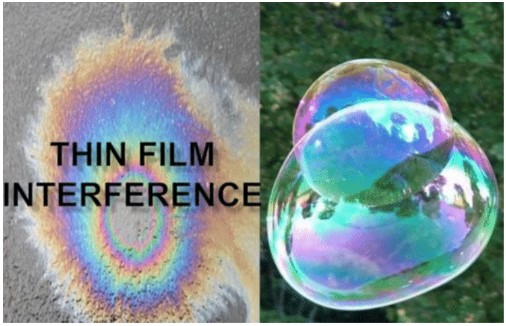
- Polarization: Using polaroid material, the direction of vibrating light waves can be modified from many directions to ONLY one specific direction.
- Polarizer: The material through which light get polarized (polarizing filter)
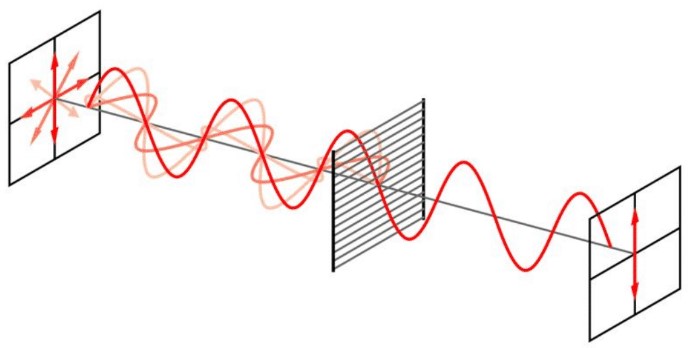
remaining today
remaining today