- What an aromatic compound is and how we can represent aromaticity.
- The properties of the important compound aromatic chemistry is based on.
- How to correctly draw and describe aromatic rings in organic chemistry.
- How to name aromatic compounds using IUPAC systematic nomenclature.
Notes:
- We learned a little bit about cyclic alkanes in Alkanes. Cyclic compounds are compounds where the carbon atoms form a ring and they are very common in organic chemistry. One of the most important cyclic compounds is benzene, which has the molecular formula C6H6.
Benzenes structure is a six-carbon cyclohexene ring with three C=C double bonds, alternating with single bonds around the hexagonal ring. So overall, each carbon atom in the ring has one C-C single bond, one C=C double bond and one C-H bond to a hydrogen atom. Its IUPAC systematic name would be 1,3,5-cyclohexatriene (or cyclohexa-1,3,5-triene). - The evidence from studying benzene shows that it doesnt actually have C=C double bonds and C-C single bonds alternating in a ring. The bond lengths show the bonds to be more like single and a half bonds.
- The other evidence shows benzene to be more resistant to reaction than alkenes. We saw in Alkenes and unsaturated hydrocarbons C=C double bond can be opened up in an addition reaction by a few different reactants. This is not true when it comes to the double bonds of benzene.
- We can draw the delocalised model of benzene: a circular ring inside the hexagon, with no double bonds. This is the aromatic ring made up of the delocalised electrons.
- We can draw two resonance structures of benzene: two different structures with the double bonds in the opposite places to each other. The idea is that the real structure of benzene is an average of these two structures. Imagine black and white being drawn to represent the colour grey which somebody has never seen before. Important: Benzene is NOT constantly jumping between the two resonance forms.
- Aromatic rings are much more stable than alkenes because the electrons that form and break bonds are not stuck in one place between two particular atoms. The electrons are delocalized throughout a larger area; here it is the six-carbon ring.
- The reason regular alkenes are more reactive is because the pair of electrons is concentrated between two atoms. It is an area of high electron density, so anything that needs electrons will react there.
It is harder for bonds to break/form if there is no focal point (where it is very electron rich or poor) for the electrons to move in and out of. - Naming aromatic compounds is not clear-cut because many old names of compounds, from before IUPAC systematic naming was created, are still popular and accepted today – "preferred" and systematic names both exist and are considered correct. Also, an aromatic compound can be named referring to the benzene ring by the prefix "phenyl" or the suffix "-benzene". A general guide that provides correct systematic names can be used below: Remember phenyl substituents.
- Treat benzene as a 6 member alkene carbon chain.
- Treat all groups below alkenes as lower priority, using the suffix –benzene if these are the only groups present in the molecule.
- Treat all carbon chains longer than 6 carbons or groups higher than alkenes as higher priority, using the prefix phenyl- for these compounds.
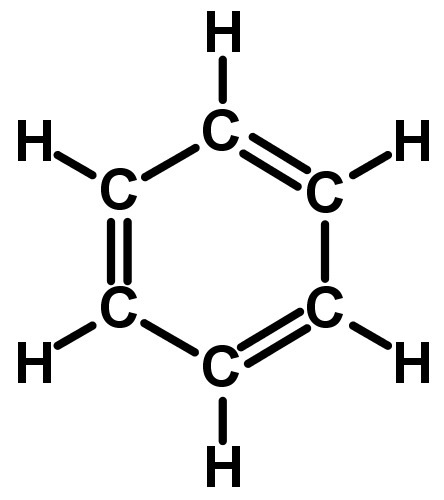
The reason we dont call it cyclohexatriene is because benzene doesnt have alkene properties, so it shouldnt be considered an alkene.
You can learn about this in more detail in Structure, bonding and reactions of benzene.
With benzene, it is more accurate to say the three pairs of double bond electrons are delocalized throughout the ring, which makes the whole ring containing these bonds resistant to chemical reactions.
This property is called aromaticity, the ring is called an aromatic ring and compounds that contain them are aromatic compounds. Benzene is therefore not an alkene, it is an aromatic compound.
The problem with benzenes aromatic properties is that when we show the structure, regular single and double bonds do not accurately describe it. Its better to think of the bonds as single and a half. We have two different ways to accurately draw the structure of benzene:
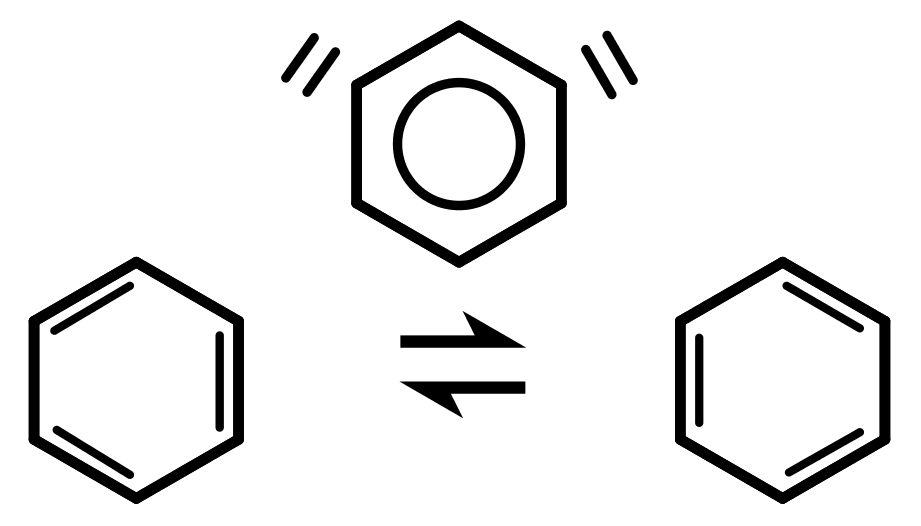
The ring substituents (the atoms bonded to the ring carbons, e.g. the hydrogens in benzene) can be substituted, but this is quite tough to do. This is because the reactions often have to break up the delocalised electron system made by the ring (the aromaticity). Aromatic rings are therefore very important in chemical reactions they will probably need to be broken for any reaction to occur.