Transformers and power transmission
Intros
Examples
Lessons
- An ideal transformer with 120V, ac supply, on the primary coil supplies power to the resistor R. If this resistor dissipates 35W, what is the current in the primary coil and the secondary coil?
- The secondary coil in an ideal transformer has 5 times as many windings as the primary. If the current in the primary is 0.40A, determine the current in the secondary, and the type of the transformer.
- A certain step-down transformer has a 500-turns primary that operates at 120V ac supply. Which one of the following sets of conditions could describe the secondary turns and voltages of this transformer?
- A step-down transformer is required to operate a 12 V, 25 W halogen lamp. Which of the following sets of conditions could apply to this transformer?
- Np=20,Ns=200
- Vp=120V,Is=0.21A
- Ip=2.1A,Is=2.1A
- Vp=120V,Ip=0.21A
- An ideal transformer has a potential difference of 130V ac across the primary windings and a potential difference of 780V ac across the secondary windings. There are 390 turns in the secondary. The secondary current is
- twice the primary current
- one half the primary current
- six times the primary current
- one-sixth the primary current
Free to Join!
Easily See Your Progress
We track the progress you've made on a topic so you know what you've done. From the course view you can easily see what topics have what and the progress you've made on them. Fill the rings to completely master that section or mouse over the icon to see more details.Make Use of Our Learning Aids
Earn Achievements as You Learn
Make the most of your time as you use StudyPug to help you achieve your goals. Earn fun little badges the more you watch, practice, and use our service.Create and Customize Your Avatar
Play with our fun little avatar builder to create and customize your own avatar on StudyPug. Choose your face, eye colour, hair colour and style, and background. Unlock more options the more you use StudyPug.
Topic Notes
Introduction to Transformers and Power Transmission
Transformers play a crucial role in power transmission and electrical systems. The introduction video provides a comprehensive overview of these essential devices, offering valuable insights into their functionality and significance. Transformers are electromagnetic devices that transfer electrical energy between two or more circuits through electromagnetic induction. They are fundamental components in power distribution networks, enabling the efficient transmission of electricity over long distances. By stepping up or stepping down voltage levels, transformers ensure that power can be transmitted with minimal losses and distributed safely to end-users. In electrical systems, transformers serve various purposes, including voltage regulation, isolation, and impedance matching. Their ability to change voltage levels makes them indispensable in both residential and industrial applications. Understanding transformers is crucial for anyone involved in electrical engineering or interested in the intricacies of power transmission. The video introduction serves as an excellent starting point for grasping the basic principles and importance of transformers in modern electrical infrastructure.
Understanding Transformers: Function and Components
Transformers are essential electrical devices that play a crucial role in our modern world. Their primary function is to change the voltage of alternating current (AC) electricity, either increasing it (step-up) or decreasing it (step-down). This ability to manipulate voltage levels is fundamental to the efficient transmission and distribution of electrical power.
At the heart of a transformer are two main components: the primary coil and the secondary coil. These coils are made of insulated wire and are wound around a common iron core. The primary coil is connected to the input AC power source, while the secondary coil is connected to the output or load. The iron core serves as a magnetic link between these two coils, facilitating the transfer of energy.
The transformer's operation is based on the principle of electromagnetic induction. When AC flows through the primary coil, it creates a changing magnetic field in the iron core. This fluctuating magnetic field then induces a voltage in the secondary coil. The ratio of turns in the primary coil to the secondary coil determines whether the transformer steps up or steps down the voltage.
For instance, if the secondary coil has more turns than the primary coil, the output voltage will be higher than the input voltage, creating a step-up transformer. Conversely, if the secondary coil has fewer turns, the output voltage will be lower, resulting in a step-down transformer. This relationship between the number of turns and voltage is crucial for the transformer's functionality.
Transformers are ubiquitous in our daily lives, often operating silently in the background. One common application is in television sets, where transformers help convert the high voltage from power outlets to the lower voltages required by various components within the TV. Another everyday example is the large transformers you see on utility poles. These step-down transformers reduce the high voltage used for long-distance power transmission to the lower voltage suitable for household use.
It's important to note that transformers only work with alternating current (AC) voltage. This is because the transformer's operation relies on the changing magnetic field produced by AC. Direct current (DC) cannot create this fluctuating magnetic field, making transformers ineffective with DC power sources. This is why AC is the standard for power distribution in most countries.
The reason transformers are so crucial with AC power is their ability to efficiently change voltage levels. High voltage is ideal for long-distance power transmission as it reduces energy loss. However, this high voltage is dangerous and impractical for household and most industrial uses. Transformers allow for the safe and efficient step-down of voltage at various points in the power distribution network.
In addition to power distribution, transformers find applications in many other areas. They are used in electronic devices to isolate circuits, in audio equipment to match impedances, and in welding machines to provide the high currents needed. The versatility of transformers makes them indispensable in modern electrical and electronic systems.
Understanding the function and components of transformers provides insight into how our electrical infrastructure operates. From the massive transformers in power stations to the tiny ones in our smartphones, these devices are fundamental to the reliable and safe use of electricity in our daily lives. As technology advances, the principles of transformer operation continue to be applied in new and innovative ways, ensuring their relevance well into the future.
Types of Transformers: Step-Up and Step-Down
Transformers are essential components in electrical power systems, playing a crucial role in voltage conversion. The two main types of transformers are step-up and step-down transformers, each serving distinct purposes in power distribution networks.
Step-up transformers, as the name suggests, increase voltage levels. These transformers have more turns in the secondary coil compared to the primary coil. This configuration results in a higher output voltage than the input voltage. For example, a step-up transformer might take 240V from a power plant and increase it to 400,000V for long-distance transmission. Step-up transformers are primarily used at power generation stations to boost voltage for efficient long-distance power transmission through power lines.
On the other hand, step-down transformers decrease voltage levels. They have fewer turns in the secondary coil compared to the primary coil, resulting in a lower output voltage. For instance, a step-down transformer might reduce 400,000V from transmission lines to 240V for residential use. These transformers are commonly found in substations and on utility poles, preparing electricity for safe consumption in homes and businesses.
The key difference between step-up and step-down transformers lies in their coil turn ratios. In a step-up transformer, the secondary coil has more turns than the primary, while in a step-down transformer, the primary coil has more turns than the secondary. This difference in coil turns directly affects the voltage output.
To illustrate, imagine a step-up transformer with 100 turns in the primary coil and 1000 turns in the secondary coil. If the input voltage is 120V, the output voltage would be 1200V. Conversely, a step-down transformer might have 1000 turns in the primary coil and 100 turns in the secondary. With an input of 1200V, it would produce an output of 120V.
In power systems, step-up transformers are typically used at the beginning of transmission lines. They increase voltage to minimize power losses during long-distance transmission. Step-down transformers are used at various points along the power distribution network, gradually reducing voltage to levels suitable for end-users. This system of voltage conversion ensures efficient power transmission and safe electricity usage in our daily lives.
Understanding the differences between step-up and step-down transformers is crucial for electrical engineers and technicians working with power lines transformers. These devices are fundamental to the operation of our electrical grid, enabling the efficient and safe distribution of power from generation sources to consumers.
Transformer Equations and Principles
Transformers are essential components in electrical systems, enabling the efficient transfer of electrical energy between circuits. Understanding the key equations and principles governing transformer operation is crucial for engineers and technicians working with power systems. This section will explore the fundamental transformer equations, including the voltage and turns ratios, as well as Faraday's law and its application to transformers.
The primary equation governing transformer operation is the turns ratio equation, which relates the number of turns in the primary and secondary windings to their respective voltages:
Vp / Vs = Np / Ns
Where Vp is the primary voltage, Vs is the secondary voltage, Np is the number of turns in the primary winding, and Ns is the number of turns in the secondary winding. This equation demonstrates that the ratio of voltages is equal to the ratio of turns in an ideal transformer.
Another crucial equation is the current ratio equation, which is inversely proportional to the turns ratio:
Ip / Is = Ns / Np
Where Ip is the primary current and Is is the secondary current. This relationship ensures that power is conserved in the transformer, as the product of voltage and current remains constant (ignoring losses).
Faraday's law of electromagnetic induction is the underlying principle behind transformer operation. It states that the induced electromotive force (EMF) in a circuit is proportional to the rate of change of magnetic flux through the circuit. In transformer terms, this can be expressed as:
E = -N * (dΦ/dt)
Where E is the induced EMF, N is the number of turns in the winding, and dΦ/dt is the rate of change of magnetic flux. This equation explains how voltage is induced in the secondary winding of a transformer.
Applying Faraday's law to transformers, we can derive the voltage equation for both primary and secondary windings:
Vp = -Np * (dΦ/dt)
Vs = -Ns * (dΦ/dt)
These equations show that the induced voltage in each winding is proportional to the number of turns and the rate of change of magnetic flux.
To illustrate the practical application of these equations, let's consider an example. Suppose we have a transformer with 1000 turns in the primary winding and 250 turns in the secondary winding. If the primary voltage is 240V, what is the secondary voltage?
Using the turns ratio equation:
Vp / Vs = Np / Ns
240 / Vs = 1000 / 250
Vs = 240 * (250 / 1000) = 60V
Therefore, the secondary voltage is 60V.
Another important aspect of transformer calculations is power transfer. In an ideal transformer, the power in the primary winding equals the power in the secondary winding:
Pp = Ps
Vp * Ip = Vs * Is
This equation is useful for determining currents when voltages and one current are known. For instance, if we know the primary voltage is
Power Transmission and Transformers
Transformers play a crucial role in power transmission systems, enabling the efficient transfer of electrical energy over long distances. These devices are essential components in the power grid, allowing for the conversion of voltage levels to minimize power losses and ensure safe, reliable electricity delivery to consumers. Understanding the function of transformers in transmission power systems is key to grasping the complexities of modern electrical infrastructure.
One of the primary reasons for using high voltages in long-distance power transmission is to reduce power losses. As electricity travels through power lines, some energy is inevitably lost due to the resistance of the conductors. This loss is proportional to the square of the current flowing through the lines. By increasing the voltage and correspondingly decreasing the current, transmission companies can significantly reduce these losses, making the process more efficient and cost-effective.
Transformers enable this high-voltage transmission by stepping up the voltage at the power plant before it enters the transmission lines. At the receiving end, near population centers or industrial areas, other transformers step down the voltage to levels suitable for local distribution and consumption. This system of step-up and step-down transformers is what makes long-distance power transmission feasible and efficient.
To illustrate the importance of high-voltage transmission, let's consider an example of power loss calculations at different voltages. Imagine we need to transmit 100 MW of power over a distance of 100 km. If we were to transmit this power at 11 kV, which is a typical distribution voltage, the current in the line would be extremely high, resulting in significant power losses. The power loss in this scenario could be as high as 20% or more of the total power transmitted.
However, if we use transformers to step up the voltage to 400 kV for transmission, the current in the line would be much lower. With the same amount of power being transmitted, the power loss at 400 kV might only be around 2-3% of the total power. This dramatic reduction in power loss demonstrates why high-voltage transmission lines and transformers are so important in modern power systems.
Power lines transformers not only facilitate this voltage conversion but also provide other benefits to the transmission system. They help in maintaining voltage stability, isolating different sections of the grid, and providing a means to control power flow. Transmission transformers are specially designed to handle high voltages and large power capacities, often incorporating advanced cooling systems and protective measures to ensure reliable operation.
The efficiency of power transmission is further enhanced by the use of three-phase systems, which are more economical than single-phase systems for long-distance transmission. Transformers in these systems are typically configured in a delta or wye (star) arrangement, allowing for flexible voltage transformations and improved system stability.
Despite the efficiency gains provided by high-voltage transmission and transformers, some power loss is still inevitable. Transmission lines experience both resistive losses (I²R losses) and corona losses, especially at very high voltages. Transformers themselves are not 100% efficient, with small losses occurring due to core losses (hysteresis and eddy currents) and copper losses in the windings.
To minimize these losses, power companies employ various strategies. These include using high-quality conductors with lower resistance, optimizing the design of transmission towers to reduce corona effects, and implementing advanced transformer technologies such as amorphous core materials and superconducting windings.
In conclusion, transformers are indispensable in power transmission systems, enabling the use of high voltages that significantly reduce power losses over long distances. By facilitating the efficient transfer of electrical energy from generation sites to consumers, transformers play a vital role in maintaining a reliable and cost-effective power supply. As technology advances, ongoing improvements in transformer design and transmission systems continue to enhance the efficiency and reliability of our electrical infrastructure, ensuring that power reaches its destination with minimal loss and maximum effectiveness.
Efficiency and Challenges in Power Transmission
The efficiency of transformers and power transmission systems plays a crucial role in the modern electrical grid. As our energy demands continue to grow, so does the importance of optimizing these systems to minimize power loss and maximize transmission efficiency. Long-distance power transmission presents unique challenges that engineers and researchers are constantly working to overcome.
Transformers are essential components in power transmission, allowing for the efficient transfer of electricity across vast distances. These devices step up voltage for long-distance transmission and step it down for local distribution. Modern transformer technology has made significant strides in improving efficiency, with high-performance materials and advanced designs reducing energy losses. Amorphous metal cores and superconducting transformers are examples of innovations that have dramatically increased transformer efficiency.
One of the primary challenges in long-distance power transmission is the issue of power loss. As electricity travels through transmission lines, some energy is lost due to resistance in the conductors. This loss increases with distance, making it a significant concern for large-scale power grids. To combat this, utilities employ various strategies for power loss reduction. High-voltage direct current (HVDC) transmission systems have become increasingly popular for long-distance power transfer, as they experience lower losses compared to alternating current (AC) systems over great distances.
The development of smart grid technology has revolutionized power transmission systems. Smart grids utilize advanced sensors, communication networks, and data analytics to monitor and control the flow of electricity in real-time. This enhanced visibility and control allow for more efficient power distribution, quicker response to outages, and better integration of renewable energy sources. Smart grid systems also enable demand response programs, where consumers can adjust their energy usage based on grid conditions, further improving overall system efficiency.
Balancing efficiency, safety, and cost is a constant challenge in power transmission. While highly efficient systems may reduce operational costs in the long run, they often require significant upfront investments. Safety considerations, such as protection against electromagnetic fields and cybersecurity measures for smart grid systems, add another layer of complexity to the equation. Utilities and regulators must carefully weigh these factors to ensure a reliable and cost-effective power supply.
Recent advancements in transformer technology have focused on not only improving efficiency but also reducing environmental impact. Dry-type transformers, which use air for cooling instead of oil, are becoming more common in urban areas due to their reduced fire risk and environmental benefits. Additionally, the development of biodegradable transformer oils offers a more eco-friendly alternative to traditional mineral oils.
The integration of renewable energy sources into the power grid presents both opportunities and challenges for transmission systems. While renewables help reduce carbon emissions, their intermittent nature requires more flexible and responsive grid management. Energy storage systems, such as large-scale batteries and pumped hydro storage, are increasingly being used to balance supply and demand, further enhancing grid stability and efficiency.
As we look to the future, the continued evolution of power transmission systems will be vital in meeting growing energy demands while minimizing environmental impact. Research into ultra-high voltage transmission, superconducting materials, and advanced grid management algorithms promises to push the boundaries of what's possible in terms of transmission efficiency. By embracing these technologies and continuing to innovate, we can build a more resilient, efficient, and sustainable power infrastructure for generations to come.
Conclusion: The Future of Transformers in Power Systems
Transformers play a crucial role in power transmission, serving as the backbone of our electrical grid. As demonstrated in the introductory video, these devices efficiently step voltage up or down, enabling long-distance power distribution. Understanding transformer technology is essential for grasping the complexities of modern power systems. Looking ahead, the future of transformer technology is bright, with advancements in materials science and smart grid integration promising even greater efficiency and reliability. Innovations like solid-state transformers and high-temperature superconducting materials are set to revolutionize power transmission. These developments will be critical in meeting the growing energy demands of our increasingly electrified world. As we move towards a more sustainable future, transformers will continue to evolve, adapting to new energy sources and grid configurations. To stay informed about these exciting developments, we encourage you to explore further resources on transformer technology and power systems. Your engagement with this topic could shape the future of energy distribution.
Introduction to Transformers
Transformers are essential devices in the field of electrical engineering, particularly in power transmission. They play a crucial role in converting voltages to meet different requirements. This guide will walk you through the fundamental concepts of transformers, their types, and their applications.
Step 1: Understanding the Purpose of Transformers
Transformers are devices used to increase or decrease voltage levels. Depending on the requirement, transformers can convert low voltage to high voltage or vice versa. For instance, in a TV set, transformers are used to provide high voltages necessary for the picture tube. When you plug your TV into an electric socket, the internal transformers convert the low voltage from the socket to the high voltage needed by the picture tube.
Another example is the utility poles that use transformers to reduce the high voltage from the electricity company to a usable voltage for household appliances. Power stations may generate electricity at voltages as high as 12,000 volts, but household devices typically require around 240 volts or 120 volts. Transformers are used to step down this high voltage to a safer, usable level.
Step 2: AC Voltage and Transformers
Transformers operate exclusively with alternating current (AC) voltage. Direct current (DC) voltage does not induce any change in magnetic flux, which is necessary to generate an electromotive force (EMF). When the direction of the current changes in an AC supply, it induces an EMF and a corresponding current. This induced EMF is crucial for the operation of transformers. Therefore, connecting a transformer to a DC supply will not work; it must be connected to an AC supply.
Step 3: Components of a Transformer
A transformer consists of two main coils: the primary coil and the secondary coil. The primary coil is connected to the input voltage source, while the secondary coil is connected to the output device that requires the transformed voltage. These coils are interconnected through an iron core, which facilitates the transfer of the induced EMF from the primary coil to the secondary coil.
The iron core plays a vital role in ensuring that the magnetic flux generated in the primary coil is efficiently transferred to the secondary coil. In an ideal transformer, it is assumed that all the magnetic flux is transferred from the primary to the secondary coil, resulting in high efficiency, typically around 90% to 98%.
Step 4: Working Principle of Transformers
When the primary coil is connected to an AC supply, it generates a changing magnetic flux. This changing flux induces an EMF in the secondary coil. The voltage induced in the secondary coil depends on the number of turns or coils in the secondary winding compared to the primary winding. If the secondary coil has fewer turns than the primary coil, the voltage will be lower. Conversely, if the secondary coil has more turns, the voltage will be higher.
The device connected to the secondary coil will receive either a higher or lower voltage based on the transformer's design. This principle allows transformers to be used in various applications, from household appliances to large-scale power transmission systems.
Step 5: Reducing Power Loss in Transmission Lines
One of the significant applications of transformers is in reducing power loss during electricity transmission. High voltage transmission is more efficient as it reduces the current flowing through the transmission lines, thereby minimizing resistive losses. Transformers are used to step up the voltage for long-distance transmission and then step it down for safe distribution to homes and businesses.
By using transformers to manage voltage levels, power companies can ensure that electricity is transmitted efficiently over long distances, reducing energy loss and improving the overall reliability of the power grid.
FAQs
-
What is the transmission of power?
Power transmission refers to the process of transferring electrical energy from power generation plants to substations near populated areas. This is typically done using high-voltage transmission lines to minimize power losses over long distances. Transformers play a crucial role in this process by stepping up the voltage for transmission and then stepping it down for distribution to end-users.
-
What is a transformer and how does it work?
A transformer is an electrical device that transfers energy between two circuits through electromagnetic induction. It consists of two or more coils of wire wound around a core, typically made of iron. When alternating current flows through the primary coil, it creates a changing magnetic field in the core, which induces a voltage in the secondary coil. The ratio of turns in the primary and secondary coils determines whether the transformer steps up or steps down the voltage.
-
What are the 3 types of transformers?
The three main types of transformers are:
- Step-up transformers: Increase voltage for long-distance transmission
- Step-down transformers: Decrease voltage for local distribution
- Isolation transformers: Maintain the same voltage but provide electrical isolation between circuits
Each type serves specific purposes in power systems and electrical applications.
-
How do transformers work in the power grid?
In the power grid, transformers are used at various stages:
- At power plants, step-up transformers increase voltage for efficient long-distance transmission.
- At substations, step-down transformers reduce voltage for regional distribution.
- On utility poles or in local areas, distribution transformers further reduce voltage for residential and commercial use.
This system of transformers allows for efficient power transmission and safe distribution to end-users.
-
How do transformers reduce transmission loss?
Transformers reduce transmission loss by stepping up the voltage for long-distance transmission. Higher voltage means lower current for the same amount of power. Since power loss in transmission lines is proportional to the square of the current (I²R losses), reducing the current significantly decreases power loss. For example, transmitting power at 400 kV instead of 11 kV can reduce losses from over 20% to just 2-3% over the same distance.
Prerequisite Topics
Understanding the fundamental concepts that lay the groundwork for more advanced topics is crucial in any field of study, and this is especially true when it comes to transformers and power transmission. While there are no specific prerequisite topics provided for this subject, it's important to recognize that a strong foundation in basic electrical principles and physics is essential for grasping the complexities of transformers and power transmission systems.
To fully comprehend transformers and power transmission, students should have a solid understanding of electrical circuits, magnetism, and electromagnetic induction. These foundational concepts form the basis for how transformers function and how electrical power is transmitted over long distances. Without this knowledge, it can be challenging to grasp the intricacies of transformer design, operation, and their role in power distribution networks.
Additionally, a basic understanding of alternating current (AC) and direct current (DC) is crucial. Transformers primarily work with AC power, and understanding the characteristics of AC circuits is vital for comprehending how transformers step voltage up or down. This knowledge also helps in understanding why AC is preferred for long-distance power transmission over DC.
Mathematics, particularly trigonometry and complex numbers, plays a significant role in analyzing transformer behavior and power transmission systems. These mathematical tools are essential for calculating power factors, phase angles, and other important parameters in electrical systems.
Furthermore, a basic grasp of materials science can be beneficial, as it helps in understanding the properties of core materials used in transformers and the conductors used in power transmission lines. This knowledge aids in comprehending efficiency considerations and loss reduction techniques in both transformers and transmission systems.
While not explicitly listed as prerequisites, familiarity with electrical safety principles is also crucial when studying transformers and power transmission. These systems deal with high voltages and currents, and understanding the associated risks and safety measures is paramount for anyone working in this field.
By building a strong foundation in these related areas, students will find themselves better equipped to tackle the complexities of transformers and power transmission systems. This comprehensive understanding not only facilitates easier learning of advanced concepts but also enables a deeper appreciation of the intricate workings of our modern electrical infrastructure.
In conclusion, while specific prerequisites may not be listed, the importance of a well-rounded understanding of electrical fundamentals cannot be overstated when approaching the study of transformers and power transmission. This knowledge serves as the building blocks for more advanced concepts and ensures a more thorough and meaningful learning experience in this critical area of electrical engineering.
In this lesson, we will learn:
- What does a transformer do and what does it consist of?
- Types of transformers
- Transformers equations
- How to reduce power loss in transmission lines?
Notes:
- A device for increasing or decreasing an ac voltage.
- Example of transformers:
- There is a transformer in TV sets to provide high voltage for the picture tube.
- Utility poles require transformers to reduce the high voltage from the electric company to a useable voltage in houses.
- Transformers operate only with ac voltages; dc voltage does not produce any change in flux to induce an emf.
- A transformer consists of two coils, the primary coil, and a secondary coil.
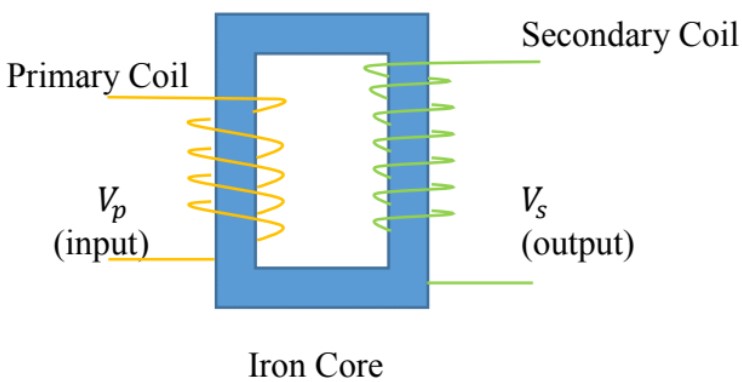
- The two coils are interconnected with an iron core.
- Nearly all magnetic flux produced in the primary coil is transferred to the secondary coil.
- As the primary coil is connected to an ac voltage, the change in the magnetic field induced a current, which is then transferred to the secondary coil with the same frequency.
- Due to the number of coils, the voltage would be different in the secondary coil compared to that of the primary.
According to Faraday’s law, the voltage induced in the secondary coil can be calculated using the following equation:
Ns= number of turns in the secondary coil
ΔtΔϕB= the rate of change in flux
The input voltage would be the result of the change in flux in the primary coil, therefore;
Np= number of turns in the primary coil
Assuming no or little amount of flux is lost, we divide the above two equations;
The above equation shows that the voltage in the secondary coil is related to the primary coil.
There are two types of transformer:
- Step-up transformer
If the number of turns in the secondary coil is more than the primary coil; the voltage in the secondary coil would be higher than that of the primary.Ns > Np⇒Vs > Vp - Step- down transformer
If the number of turns in the primary coil is more than the secondary coil; the voltage in the primary coil would be higher than that of the secondary.Np > Ns⇒Vp > Vs
Note: the output power is equal to the input power in ideal transforms where their efficiency is 99%.
Ps=IsVs
Pp=IpVp
- Transformers are very important electric devices in transmitting electricity from the power plant to houses.
- Since the power has to be transmitted over long distances, part of the energy will be lost in the transmission lines.
- To minimize the loss in energy, the power is transmitted at high voltage.
- This is done by
- Using step-up transformers to increase the voltage, as the power leaves the power plant station.
- Using step-down transformers to decrease the voltage before the power enters the houses.
Example of Transmission Lines
An average of 140 KW of electric power is sent from a power plant, 20 km away, to a small town. The total resistance of the transmission lines is 0.60Ω. calculate the power loss if the power is transmitted at:
- 240V
- 12000 V
Solution
- To find the power loss we should apply the equation P=I2R,
- The equation P=RV2 does not work in this case, since we do not have the voltage drop across the lines.
- Step 1
calculate the current:
I=VP=2.4×1021.4×105= 583.3 A
Step 2
Find the power loss:
P=I2R=(583.3)2(0.60) = 204143.33 W = 204KW
Almost 60% of all overpower is lost in the power lines!!!!! - If the transmission voltage increases to 12000V,
I=VP=1.2×1041.4×105= 11.6 A≃ 12 A
P=I2R= (12)2(0.60) = 86.4W
the power loss decreases to 0.6%
remaining today
remaining today