In this lesson, we will learn:
- To recall Le Chatelier's principle when studying equilibria.
- How to predict changes to equilibria given changes in reaction conditions.
- How to explain changes in equilibrium position due to changes in reaction conditions.
Notes:
- Continuing from Dynamic Equilibrium, when more chemical reactions were studied, and more equilibria were found, chemists started changing the conditions (such as temperature and pressure) of the reaction to see what this did to the equilibrium they saw.
This led to them finding Le Chatelier's principle:- When a reaction at equilibrium is disturbed by a change in conditions, the system will respond in a way that COUNTERACTS the disturbance and then re-establish a new equilibrium.
- The system 'counteracts the disturbance' by favoring the forward or the backward reaction; it will do the opposite of whatever effect the disturbance had on the reaction! 'Favoring' a reaction means that reaction rate increases (causing a change in the ratio of reactants to products in the system), before a new equilibrium is reached as the two rates become equal again. Once this happens, the new ratio of reactant to product concentration won’t change unless equilibrium is disturbed again - in this case, the above happens again.
- Remember, 'at equilibrium' only tells us that the forward and reverse reactions are happening at the same rate. Equilibrium does not tell us how much product and reactant there is in the system!
- When a reaction at equilibrium is disturbed, it will work to re-establish equilibrium under these new conditions. If some facts about the reaction are known, the change to equilibrium position can be predicted from a change in conditions:
- If temperature is increased:
- In an exothermic reaction: The system will respond to favor the reverse reaction which leads to a greater concentration of reactants being observed in the reaction. An exothermic forward reaction, by definition, causes a net release of heat energy to the surroundings. The system therefore counteracts the disturbance of the temperature increase by temporarily favoring the reverse reaction, which will be the endothermic opposite of the exothermic forward reaction. This endothermic reverse reaction has a net absorbing of heat effect, absorbing the increase in temperature that initially disturbed the system. This is how Le Chatelier's principle works! When the reverse reaction is favored, we say the equilibrium has shifted to the left as the left-hand side of the reaction equation shows the reactants.
- In an endothermic reaction: The system will respond by favoring the forward reaction. This is for the same reasons as above; the system will favor the endothermic forward reaction, which absorbs heat and counteracts the increase in temperature that it was disturbed by. Favoring the forward reaction means the equilibrium shifts to the right. In this case, more products will be produced in the system.
- If temperature is decreased:
- In an exothermic reaction: The system will respond to favor the forward reaction. This is because the exothermic forward reaction has a net releasing of heat effect, which will raise the temperature and counteract the decrease in temperature that initially disturbed it. In this case we say the equilibrium has shifted to the right and more products will be produced.
- In an endothermic reaction: The system will respond to favor the reverse reaction. This is because the reverse reaction will be exothermic, causing a net release of heat energy to the system, raising the temperature that initially disturbed it. The equilibrium will shift to the left and more reactants will be present in the system.
- If pressure is increased, count the number of moles of gas on both sides of the reaction equation:
- When there are more moles of gas in the products than the reactants, the system will respond to favor the reverse reaction and the equilibrium will shift left. This has the effect of 'cutting back' the number of gas particles in the system as there are more of them in the products than the reactants. This leads to less gas particle collisions which decreases pressure, which counteracts the initial disturbance of a rise in pressure!
- When there are less moles of gas in the products than the reactants, the system will respond to favor the forward reaction and the equilibrium will shift right. This counteracts the disturbance in the same way as explained above.
- If pressure is decreased, count the number of moles of gas on both sides of the reaction equation:
- When there are more moles of gas in the products than the reactants, the system will respond to favor the forward reaction and the equilibrium will shift right This has the effect of increasing the number of gas particles in the system as there are more gas moles in the products than the reactants. This leads to more gas particle collisions which increases pressure, counteracting the initial disturbance of a pressure decrease!
- When there are less moles of gas in the products than the reactants, the system will favor the reverse reaction and the equilibrium will shift left. This leads to an increase in the number of gas particles in the system because there are more gas moles in the reactants than the products. This counteracts the initial disturbance of the pressure decrease.
- If a catalyst is added to the reaction mixture, there is no change to the position of equilibrium. A catalyst does not change equilibrium; it simply allows equilibrium to be reached quicker.
- The table below summarizes the changes to equilibrium position caused by a change in conditions:
- Even though chemists know how to change a reaction at equilibrium to make the largest amount of product, there are practical issues with shifting the equilibrium.
- Increasing the pressure on a closed system can be very expensive and there are safety considerations at very high pressure.
- Many reactions, such as the Haber process, have an exothermic forward reaction. This strangely means that to shift the equilibrium to make more product, you need to cool the reaction down which will reduce reaction rate.
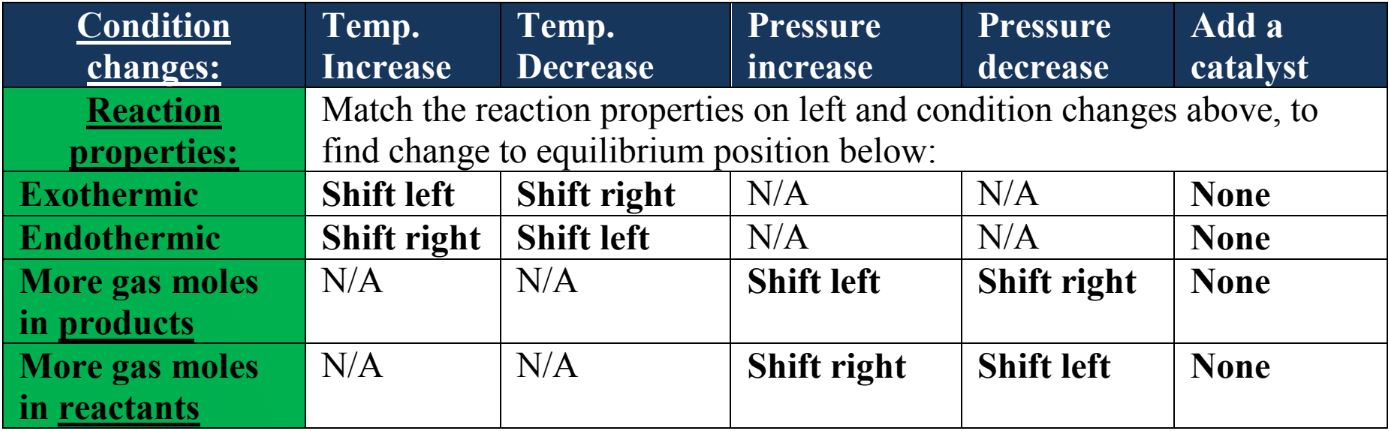